Slippery and sticky?
By Tyler Housel, Contributing Editor | TLT Lubrication Fundamentals April 2025
A lubricant must have a dual nature to establish a plane of slipperiness between objects.
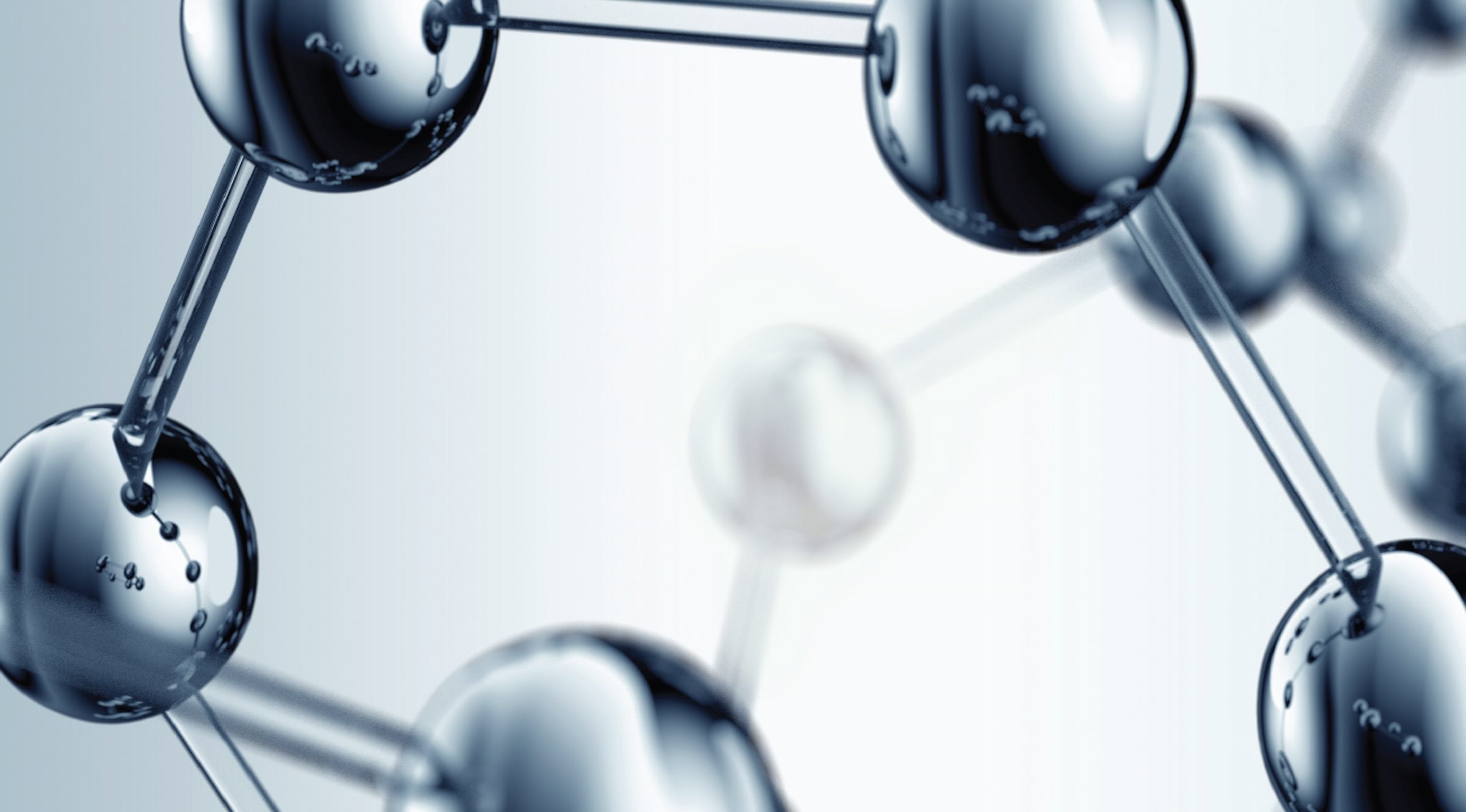
Last month, this column gave a brief explanation of the different ways that two atoms can interact to form chemical bonds. Bonding is essential to the study of chemistry and is the first step in understanding how atoms behave. To build this into a macroscopic model, we must now consider the attractive forces in three dimensions. Most atoms can bond with multiple neighbors, and the bonds often create charge separations that lead to secondary attractive forces affecting other atoms nearby. In some cases, this attraction can lock the atoms into a solid crystalline network or stabilize them in a fluid liquid state. Other times the atoms remain as distinct small molecules in the gas phase. Atomic interactions explain why each of these states exist and eventually lead to the world we experience.
As previously discussed, the Periodic Table does a great job of showing trends that govern atomic behavior. But with so many elements to choose from, which ones are the most important? Cosmologists tell us that hydrogen was the first element formed after the Big Bang and still makes up 75% of the mass in the universe. When stars form, they convert hydrogen to helium. This process releases tremendous amounts of energy because the mass of the hydrogens is slightly greater than the mass of the resulting helium nucleus, and the extra mass is converted to energy according to Einstein’s famous equation: E = mc2. Over time, the helium begins to form heavier nuclei according to the laws that govern nucleosynthesis.1 Suffice to say this process favors the formation of certain nuclei so that the present-day universe ends up with the elemental distribution shown in Table 1.2 But just remember, “There are more things in heaven and earth, Horatio, than are dreamt of in your philosophy.”3
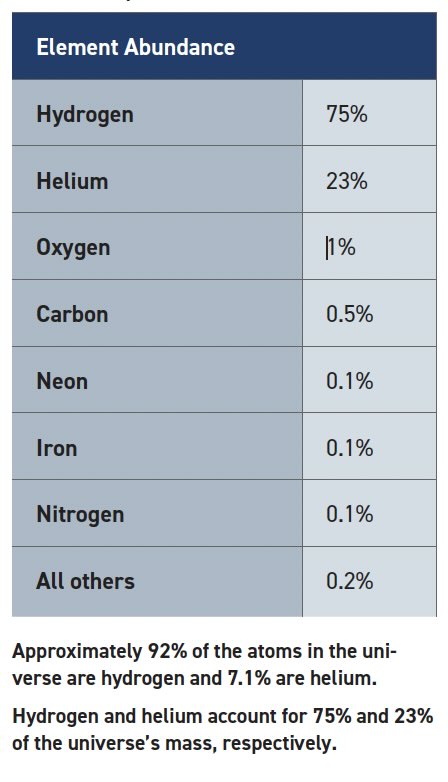
Table 1. Composition of the universe
It’s no surprise that we encounter the seven most common elements often. In fact, they make up the vast majority of Earth’s atmosphere, oceans and biomass. It seems like a lucky coincidence that a star’s nuclear reactions produce an abundance of elements that are useful for chemistry with particularly active valence electrons. After all, nucleosynthesis in stars follows completely different quantum rules than chemical reactions on Earth. Yet just four of the top seven elements give us water, hydrocarbons, alcohols, ethers, esters, amines, amides and the list goes on. What if nuclear reactions favored the conversion of hydrogen (1) and helium (2) into lithium (1 + 2 = 3) and beryllium (2 + 2 = 4)? We will never know!
It is logical to point out that stars produce the atoms by nucleosynthesis, but we don’t live on a star. Actually, there is a significant difference between the elements found in stars and what exists here on Earth (see Table 2). Oxygen is the clear winner along with silicon, aluminum and iron. But take a deep breath and ponder the fact that niobium, rubidium and yttrium are all more common than nitrogen.
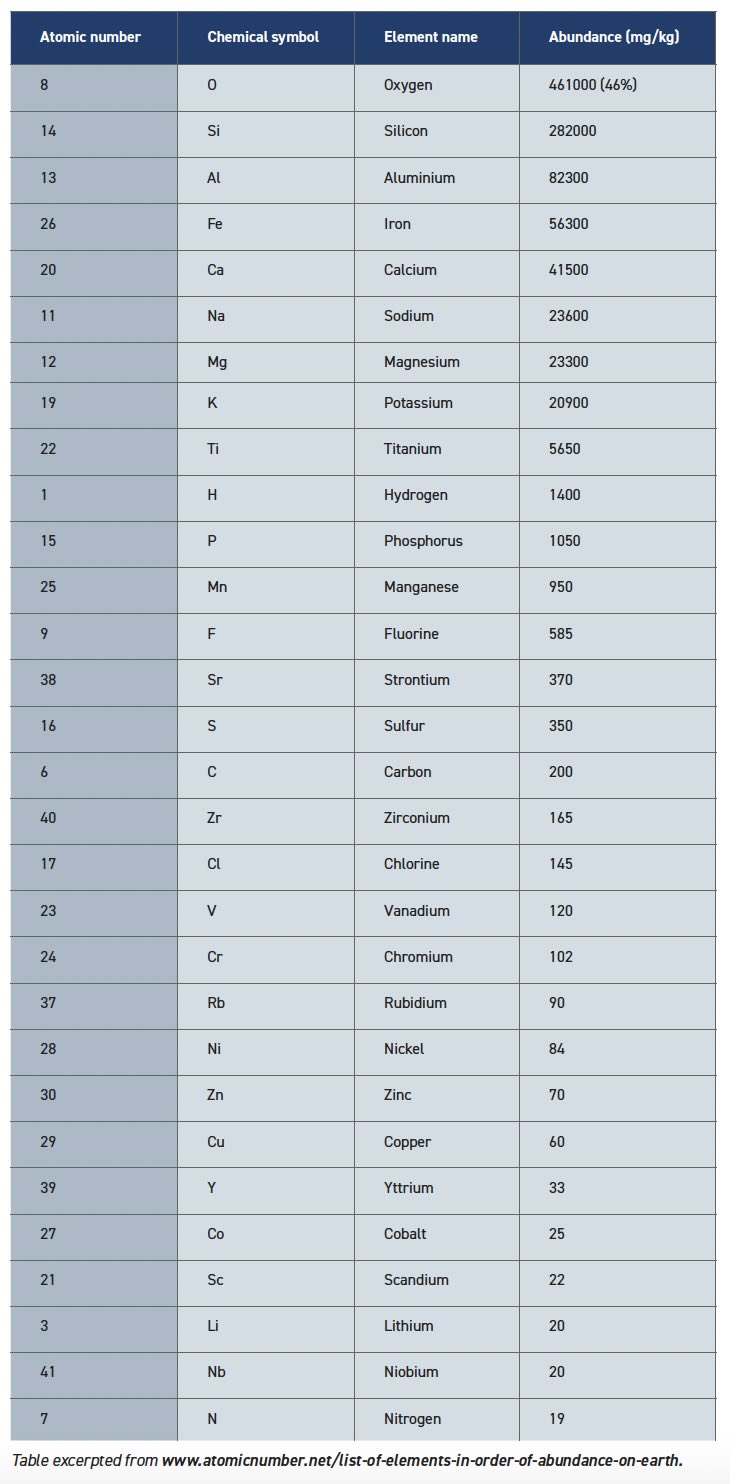
Table 2. Elements in Order of Abundance on Earth
A few years ago, I took an online course called “The Science of the Solar System” by Mike Brown of Caltech (not an STLE member, but he is the guy who demoted Pluto).4 It’s fair to ask why these particular elements ended up on Earth, and what follows is a chemist’s “how to describe it after two beers” version.
Planets form in a band of gas and dust orbiting a young star. These tiny bits bounce off each other and occasionally combine to form small particles and then continue to grow through collisions. Early on, the particles do not possess sufficient mass to rely on gravity alone to stick together. Instead, an electromagnetic attraction potential can keep these bits together as they grow. Dynamical friction also slows the relative motion of larger bodies, so collisions are less violent. Eventually, the bodies accumulate enough mass to be held together by gravity. These planetesimals continue to merge until most of the matter in the ring coalesces into one planet.
Oxygen is only 1% of the atoms in the universe, but almost half of the atoms on the surface of the Earth. Oxygen concentrates during planet formation because it is reactive and forms polar bonds with other common elements. These oxides are stable solids with a charge distribution that enhances their attraction potential, allowing oxide particles to stay together during close encounters in the vacuum of space. When gravity took over, the heavier iron and nickel sank to the core of the planet while the lighter oxides formed the crust. The elemental abundance in Table 2 demonstrates that oxygen readily combines with silicon, aluminum, iron and other metals to form three-dimensional structures, otherwise known as rocks, that dominate the landscape today.
Atoms such as helium and neon are also abundant in the universe, so they must have been in the planetary nebula, but they didn’t stick around (pun alert) because they have filled valence shells and do not form chemical bonds.5 Actually, most of the helium on Earth today comes from radioactive decay.
Tribologists and astrophysicists share more than a pompous name—we are both interested in understanding what makes things stick. Viscosity, hardness and even the state of matter are different ways to infer the strength of intermolecular attractions.
One lesson from the previous section is that helium is the most chemically inert substance in the universe, which also makes it the least sticky. At temperatures near absolute zero, helium becomes a superfluid, which is a liquid with zero viscosity.6 Before the sales team gets too excited, it’s difficult to imagine there’s a big market to lubricate machinery operating at -270°C.
Non-sticky substances are gases under ambient conditions. Noble gases are individual atoms that do not bond. Other gases like nitrogen (N2) and methane (CH4) are lightweight molecules where all of the atoms have covalently filled valence shells. These molecules are not strongly attracted to each other so that their thermal (kinetic) energy exceeds their intermolecular attraction. As planets form, thermal energy is highest near the star. Rocky planets form in the zone where oxides are solid, but the temperature is high enough to prevent gaseous molecules from condensing. In the cold outer solar system, nitrogen and methane can exist as liquids or solids on moons and other small bodies.7
Condensed phase materials (liquids and solids) have enough attractive energy to overcome their thermal energy. The attractive energy comes from either covalent bonds or intermolecular forces that chemists might recognize as hydrogen bonds, van der Waals, London, dispersion, dipole, ion pair, Brønsted-Lowry, Lewis or acid-base interactions. These names reflect different and sometimes overlapping theories to explain the intermolecular forces that can stabilize molecules in the condensed phase.
The classic example is water, which is a liquid with a molecular weight of 18. Molecules such as CH4, C2H6, C3H8, N2, O2, Ar and CO2 have molecular weights between 18 and 44, but their low polarity and weak intermolecular attraction makes them gases under ambient conditions. Water has a boiling point hundreds of degrees higher because each oxygen carries a partial negative charge and is attracted to all of the positively charged hydrogens nearby. Although the familiar formula for water is “H2O,” water acts like a large aggregate of hydrogens and oxygens because hydrogen bonds stabilize water molecules in the liquid (or solid) phase as shown (two dimensionally) in Figure 1.
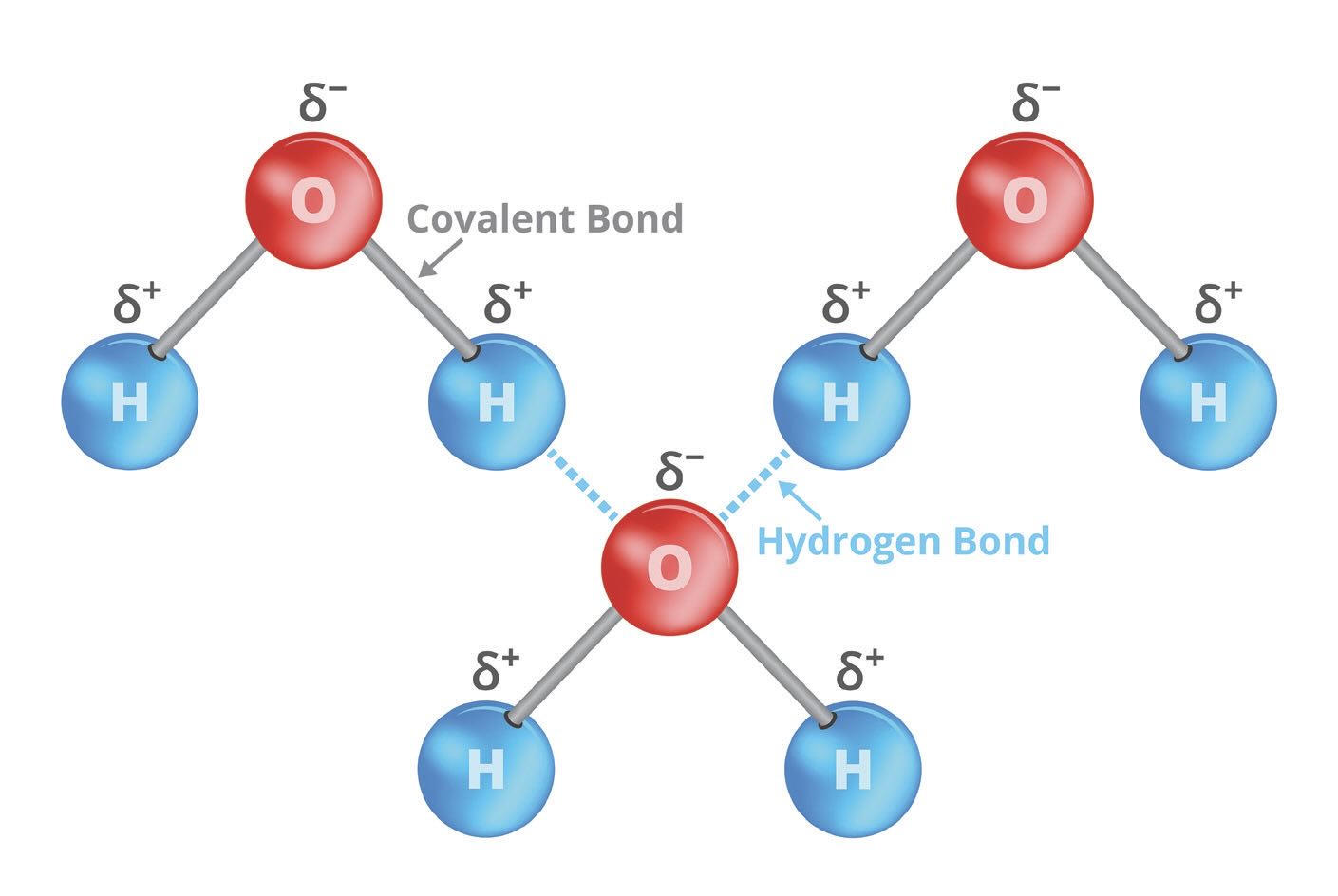
Figure 1. Vector chemical illustration of hydrogen bond or H-bond on water molecules.
Viscosity measures the flow characteristic of a fluid in response to an applied shear stress and is an excellent way to measure the internal stickiness of a liquid. The molecules in a low viscosity liquid flow over each other easily. Higher viscosity means more resistance to flow due to greater attraction between atoms. This attraction can be in the form of covalent bonds (higher molecular weight), ionic bonds (dissolved salts), hydrogen bonds (as explained earlier) or other interactions. Viscosity can even be affected by externally applied electric8 or magnetic9 fields, which modify the attractive forces between atoms in the fluid.
Figure 2 shows six types of viscosity behavior that result from different internal bond networks. Shear can affect the fluid structures as molecular motion can change the local distribution of atoms and either increase or decrease the local attractive forces relative to those in a static liquid. Higher temperature decreases viscosity because atoms with greater kinetic energy can move faster despite the attractive forces. Liquids evaporate when their thermal energy finally exceeds the attractive energy.
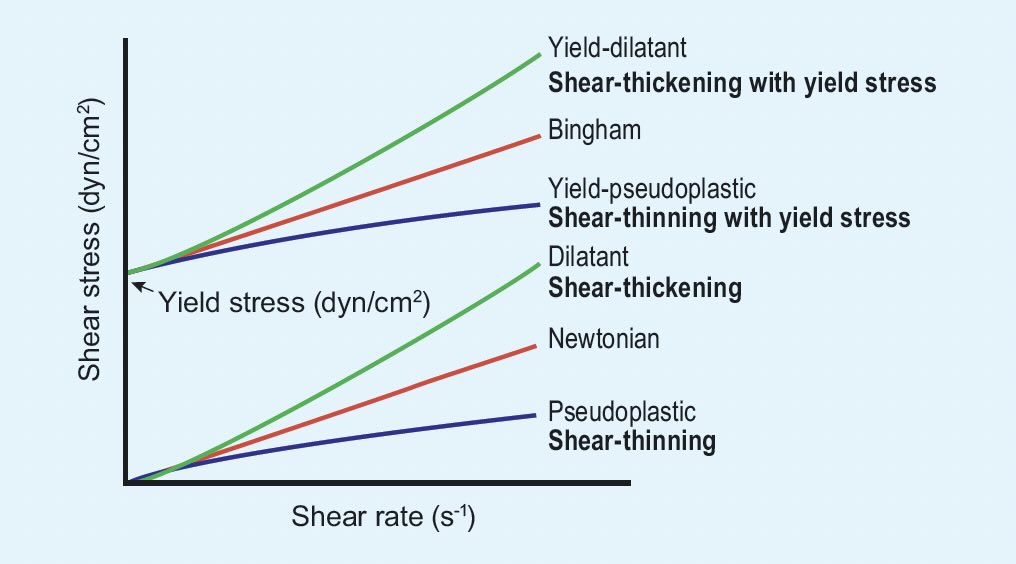
Figure 2. Rheological behaviors of Newtonian and non-Newtonian fluids. Figure courtesy of Marum, D., Afonso, M. and Ochoa, B. (2020), “Rheological behavior of a bentonite mud,” Applied Rheology, 30 (1), 107-118, https://doi.org/10.1515/arh-2020-0108.
Viscosity is a critical lubricant property and deserves a deeper treatment as the topic of a future article in TLT. In the meantime, make plans to attend the 2025 STLE Annual Meeting, May 18-22, in Atlanta, Ga. (www.stle.org/annualmeeting). Many companies will present technology and exhibit products that allow formulators to optimize viscosity and address the specific demands of any application.
Minerologists use Mohs hardness scale (see Figure 3) to compare the hardness of different minerals. There are also several scales (Rockwell, Brinell, Vickers, Knoop) that measure the hardness of metals. Hardness essentially measures the strength of attractive forces within a solid substance. If a penny scratches your three-carat engagement ring, you didn’t get the diamond you paid for.
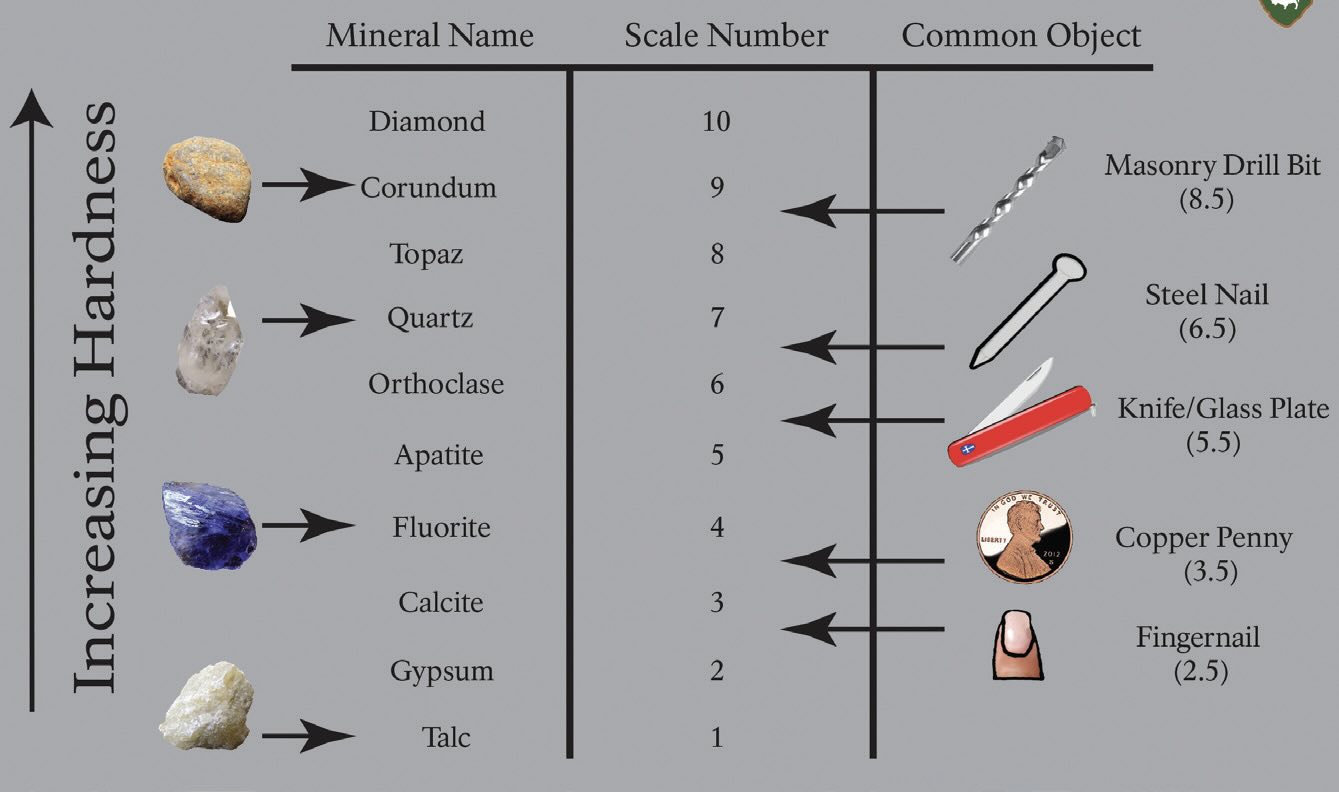
Figure 3. Mohs Hardness Scale. Figure courtesy of National Park Service, Public domain, via Wikimedia Commons.
A chain is only as strong as its weakest link, and a solid material is only as hard as the weakest bonds between atoms. Diamond crystals are hard because all of the carbons are tightly, covalently bonded to each other; there are no weak links. Meanwhile, talc is soft because it is made of thin sheets of magnesium silicate with weak bonds in between.10 Like graphite and many other dry lubricants, talc powder is slippery because the sheets slide easily against each other.
Friction is also a topic that deserves its own article. For now, just assume that a lubricant works by establishing a plane of slipperiness between two solid objects in relative motion. Since vacuum, helium and other gases are the ultimate non-sticky substances, they must be the best lubricants. But notice that there are two requirements for lubrication. Gases are non-sticky, but they cannot normally establish a plane to separate the objects we want to lubricate.
The planets use gravity to clear out their orbital path so they can travel frictionlessly through the vacuum of space. This isn’t an option on Earth, and in freshman physics, we used a “frictionless” table to study the collisions of small pucks on a glorified air hockey table. Air bearings and magnetic levitation trains are practical applications that minimize friction using air as a lubricant. Perhaps this thinking also applies to wheels and bearings. In some sense, a ball bearing is analogous to a helium atom that separates two surfaces but is not chemically attracted to either one. A car’s chassis is separated from the ground by a slippery plane of air that is established by the wheels. And safe driving requires us to maintain an air gap between the car and the objects around us.
In hydrodynamic lubrication, liquid lubricants rely on their internal stickiness to create pressure that establishes a robust, slippery plane separating the surfaces. Increasing viscosity (stickiness) increases the pressure, which increases both the thickness of lubricant layer and the internal fluid friction. The ideal viscosity is high enough to separate the surfaces but low enough to minimize this internal friction. Hydrodynamic lubrication breaks down when the combination of speed, load and temperature do not allow the lubricant to maintain a consistent film separating the two objects.
As the surfaces descend into the mixed and boundary lubrication regimes, formulators can maintain the slippery plane using additives that are attracted to the surface. Tribologists sometimes suggest that polar ingredients compete with each other as they try to bond with a limited number of metal oxides or other atoms on the surface. This chemical attraction between additive and substrate is different from internal stickiness between molecules in the fluid (viscosity).
An effective lubricant must be sticky enough to maintain a slippery plane between two moving objects so they can slide against each other with minimal friction. This means lubricants must be designed with a balance of stickiness and slipperiness! Perhaps some readers knew this from the beginning, but I was not expecting this conclusion when I started writing this article. I’ll be at the 2025 STLE Annual Meeting in May in Atlanta and look forward to hearing everyone’s thoughts.
REFERENCES
1. Johnson, J. A. (2019), “Populating the periodic table: Nucleosynthesis of the elements,” Science, 363 6426), pp. 474-478, https://doi.org/10.1126/science.aau9540.
2. https://sciencenotes.org/composition-of-the-universe-element-abundance/
3. Shakespeare, W. “Hamlet,” Act 1, Scene 5, 167-168.
4. https://mikebrown.caltech.edu/teaching/science-solar-system/lectures/week-4
5. Housel, T. (2025), “The Periodic Table,” TLT, 81 (3), pp. 30-24. Available at www.stle.org/files/TLTArchives/2025/03_March/Lubrication_Fundamentals.aspx.
6. www.scientificamerican.com/article/superfluid-can-climb-walls/
7. www.scientificamerican.com/article/methane-on-mars-titan/ and https://mashable.com/article/pluto-liquid-nitrogen-lakes
8. Kumar, M. P., Karcz, J., Kula, P., Karmakar, S. and Dhara, S. (2023), “Giant electroviscous effects in a ferroelectric nematic liquid crystal,” Phys. Rev. Applied, 19, 044082, https://doi.org/10.1103/PhysRevApplied.19.044082.
9. Doganay, S., Alsangur, R. and Turgut, A. (2019), “Effect of external magnetic field on thermal conductivity and viscosity of magnetic nanofluids: a review,” Materials Research Express, 6 (11), 112003, https://iopscience.iop.org/article/10.1088/2053-1591/ab44e9/pdf.
10. https://knowswhy.com/why-is-talc-soft/
Tyler Housel is a technologist for Hnuco Technologies and is based in Lansdale, Pa. You can reach him at tylerhousel@comcast.net.