Meet the Presenter
This article is based on a webinar titled Nanoparticle Suspensions for Energy Efficiency. Hosted by the American Society of Mechanical Engineers’ (ASME) Tribology Division and presented by STLE member Dr. Jacqueline Krim on Sept. 29, 2023, the session explored energy efficiency, climate change, friction and an overview of the macroscale and nanoscale methods used to measure tribological properties of nanosuspensions. Courtesy of STLE, this article captures the core insights from this ASME-organized event. For more technical content from the ASME Tribology Division Webinar Series, visit the ASME Tribology Division’s website at www.asme.org/get-involved/groups-sections- and-technical-divisions/technical-divisions/technical-divisions- community-pages/tribology-division.
Dr. Jacqueline Krim is a distinguished university professor emerita of physics at North Carolina State University. She received her doctorate degree in experimental condensed matter physics from the University of Washington in 1984. After a year-long appointment as a North Atlantic Treaty Organization (NATO) postdoctoral Fellow at the University d’Aix-Marseille II, France, she joined the physics department at Northeastern University at the assistant professor level. She joined North Carolina State University in 1998 as a full professor in physics, with a subsequent appointment as associate member of the Electrical and Computer Engineering Department. You can reach her at jkrim@ncsu.edu.
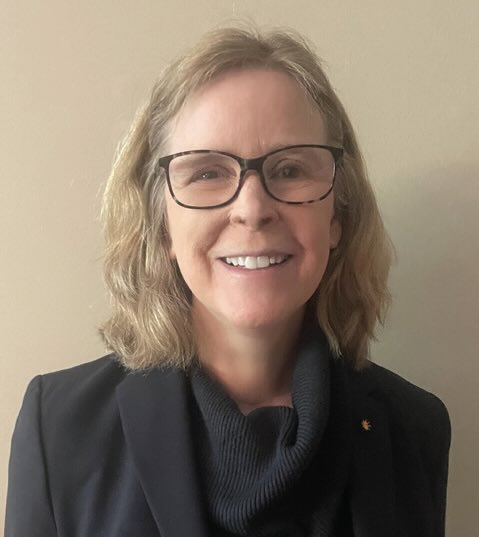
Dr. Jacqueline Krim
KEY CONCEPTS
•
Nanoparticles impact lubricant performance.
•
Macroscopic and nanoscale experimental methods can be used to measure tribology with different nanoparticle suspensions.
•
Nanoparticles can reduce friction levels and increase energy efficiency.
The objective of this article is to discuss nanoparticles’ effect on tribology, friction and lubrication. It covers five topics:
1.
Energy efficiency, climate change and friction
2.
Overview of the macroscale and nanoscale methods that we use to measure tribological properties of nanosuspensions
3.
Case study: TiO2 nanoparticles dispersed in water
4.
Case study: Nanodiamonds dispersed in base oil + additives
5.
Outlook and summary
This article is based on a webinar presented by STLE member Dr. Jacqueline Krim, a distinguished university professor emerita of physics at North Carolina State University, and the Tribology Division of the American Society of Mechanical Engineers (ASME) titled Nanoparticle Suspensions for Energy Efficiency. See Meet the Presenter for more information.
Energy efficiency, climate change and friction
Annual emissions of carbon dioxide into the atmosphere continue to increase, with about 40 gigatons of carbon dioxide emitted into the atmosphere in 2023 alone. Energy efficiency is the top component in most plans to address carbon emissions. It is defined, in simple words, as “getting the same job done but using less energy.” Friction reduction contributes to ~1/3 of these emissions reductions by increasing the fraction of useful work performed per unit of energy.
Here are key challenges in reducing friction:
•
Current lubricants encounter a range of operating conditions and are developed to work “well enough” across any environment. They therefore are not optimized for the specific operating conditions in which they are employed.
•
Lubricants that can be adjusted with fields in real time for changing conditions through tribotronic control is a challenging and highly desirable next step in optimization.
•
Testing active lubricant control requires finding a tribometer that is capable of both rapid, accurate measurements and applying a measurable and consistent field across a contact.
Nanoparticles have been studied for some time, and they are known to have a different impact in every regime of the Stribeck curve. They can also be controlled externally.
1
Nanoparticles can increase or reduce friction in test setups by very dramatic amounts. Friction reduction is of particular interest. For example, nanodiamonds can, in some cases, reduce friction by 75% or more when added to engine oil, with 3%-5% increases in fuel efficiency reported in test vehicles.
2
Charged nanoparticles can be repositioned and also provide “lift.” Charged nanoparticles are quite common. That’s because charging is one way to keep them from agglomerating. It also provides some lift if there is an electric repulsive effect, so if they are staying away from each other, they also can push these two surfaces apart and provide a lift and change the interfacial slip conditions.
Nanoparticles such as titanium dioxide aren’t just useful for engines and mechanical systems. They can also enhance performance of flow batteries in energy storage systems.
Macroscale and nanoscale methods
The study of friction’s atomic-level origins, or nanotribology, indicates that sliding friction stems from various unexpected sources, including sound energy, and static friction may arise from physisorbed molecules.3 Many methods for atomic scale friction were developed in the 1990s.
A commonly displayed Stribeck curve shows how in a macroscopic contact, the friction coefficient switches over from boundary lubrication, where the load is carried exclusively by the points of solid- solid contact, to hydrodynamic lubrication, where the load is carried exclusively by the lubricant. As speed increases, liquid starts being drawn into contact, and the friction drops. At the point of the hydrodynamic regime, the friction starts going up again and the initial part is linear with velocity.
One way to measure macroscopic friction involves a mini traction machine (MTM). Figure 1 shows MTM ball-ondisk setup. The 440SS disk is set to rotate force and sliding speed. The MTM measures the macroscopic kinetic coefficient of friction.
Figure 1. Mini traction machine (MTM). Photo courtesy of PCS Instruments.
For atomic scale measurements a quartz crystal microbalance (QCM) can be used
(see Figure 2). QCM measures interfacial friction with a fluid and also mass loading, and is particularly comparable to measurements performed at the macroscale in the hydrodynamic regime. Figure 2 shows how QCM (upper) and lubricated contacts (lower) respond to the presence of nanoparticles.
Figure 2. Quartz crystal microbalance (QCM).
A QCM is about 1-2 cm wide and vibrates at speeds where the amplitude of vibration is such that the surface is moving at macroscopic speeds (a meter/second, hundreds of cm/second). It can be immersed into a liquid and a film even 1 atom thick film can be deposited and detected.
Figure 3 shows a setup in the simplest form. Here gold quartz crystals are immersed in water, then the frequency and amplitude of vibrations are measured as nanoparticles are added.
4
Figure 3. Data recording and experimental setup employed in Ref. 4.
Figure 4 shows QCM frequency and dissipation shifts. “The original frequency is shown, oil is used and the frequency is stable, then nanoparticles (diamonds) are placed in, there is a nickel and gold surface, and after that the frequency is dropping due to mass uptake that is larger than just an increase in viscosity, but there is also an increase in resistance,” explains Krim.
•
f0: Resonant frequency of oscillator
•
df0: Reflects mass dragged along with shear motion (both fluid and adsorbed films)
•
R0: Mechanical resistance to motion. Inversely proportional to the quality factor Q
•
dR0: Reflects changes in interfacial slip conditions and viscous drag effects
Figure 4. QCM response of nanodiamonds added to oil; mass loading, abrasion, increased drag forces.5
To summarize, many systems have been studied, as an initial indication of whether friction will increase or decrease, and there are many studies that show that friction can go up or down when nanoparticles are added
(see Figure 5).
Figure 5. Nanoparticle impact in different systems.6
Krim's group studied many substrate- nanoparticle materials combinations in water and the ones that they spot checked did correctly indicate whether they would see a reduction or increase in friction at the macroscale.
6,7
Case study: TiO2 nanoparticles dispersed in water
Krim explains: “Instead of just putting one QCM into the liquid and adding nanoparticles, we are now putting a counter electrode in order to put an external field, and a reference electrode with a frit used for electrochemical measurements in order to take studies versus current versus voltage, and to move the particles around to explore the changes in friction.”
Krim explains: “3-probe cyclic voltammetry (CV) measured a positive triangle wave as is more typical in literature. CV measurements were taken to ensure the reliability of the 2-probe CV data. The slide depicts the 3-probe CV circuit, using QCMs as the working electrode (WE) and counter electrode (CE) with a nanoparticle suspension separating them and the reference electrode located directly behind the WE.”
Krim explains: “We are using positively charged aluminum nanoparticles, negatively charged TiO
2, both at about 40 nanometers diameters, and used pure water. Studying platinum, Figure 6 shows the contact angle changes significantly; it goes up for the titania and down for the alumina oxide, and there is a difference in slip length which is theoretically related to the contact angle.”
Figure 6. Compare negatively charged TiO2 nanoparticles with positively charged Al2O3 nanoparticles.8
Krim says: “When external field is applied
(see Figure 7) the alumina graph (a) has a sudden drop, particles attaching immediately without any field. Titania is very different, it is very gradual, just the background reflecting an increase in fluid density, and the resistance goes up for alumina and down for titania (b).”
Figure 7. Tuning friction and slip at solid-nanoparticle suspension interfaces by electric fields.8
Nanoparticles behave as asymmetric damped driven oscillators. Krim says: “When we look at the different systems, they are bobbing up and down at different speeds just like damped driven oscillators with very set characteristic time sets. Moving around we aren’t able to control how fast they move—they move at their pace. We could put a larger field, but in any case under this field, they bob up and down like a damped driven oscillator, and we can change the frequency driving them.”
She explains: “Figure 8 shows QCM frequency (a, b) and resistance (c, d) response versus wt for 0.67 wt% suspensions of TiO
2 and Al
2O
3 nanoparticles exposed to alternating electric fields (e, f) (100 N/C peak amplitude) oscillating at 0.005 rad/s (a, c), 0.02 rad/s (b, d, squares) and 0.3 rad/s (b, d, triangles). Shifts in opposite directions for a given bias voltage are attributed to the nanoparticles’ opposite charges. Strong asymmetry between the attractive and repulsive biases for the Al
2O
3 data is consistent with the nanoparticles residing on or near a hard wall substrate (a, c). The shape of the response for the TiO
2 data may arise from repositioning of TiO
2 nanoparticles as they are pressed into interfacial water molecules, which are not present for Al
2O
3 nanoparticles. At higher frequencies (b, d) the response diminishes, and the phase lag (d) increases.” '
Figure 8. Tuning friction and slip at solid-nanoparticle suspension interfaces by electric fields.8
In contrast, after 10 days in storage, the nanoparticle content for the TiO
2 suspensions fell below thermogravimetric analysis (TGA) sensitivity (~0.03 wt). This was also evident from a visual inspection of the vials that revealed nanoparticles settled at the bottom and a clear supernatant above. Voltammogram measurements recorded four days after the sonication (not displayed) were distinct from those recorded immediately after the sonication and were evolving one month after sonication. The voltammograms also develop new features for electric fields with periods longer than ~300 s, indicating changes in the electrochemical attributes of the system. Notably, the feature near 1 V has collapsed, confirming that it is attributable to the presence of TiO
2 nanoparticles.
9
Krim says that TiO2 reversible deposition implies a change in surface electrochemistry.
•
The feature which appears in the TiO
2 data at 300 s may arise from repositioning of TiO
2 nanoparticles as they are pressed into interfacial water molecules.
•
This mechanism is potentially an energy storing mechanism.
Krim says: “The number one question I am asked when I talk about the electro deposition and about the nano suspensions with titania is what happens to other sized particles. Smaller nanoparticles (5 nm) did not deposit, and larger nanoparticles (100 nm) did not remain in suspension.”
10
Krim summarizes: “The results reveal applications in custom design of lubricants and nanoscale energy storage systems. 40 nm diameter TiO2 nanoparticles displayed reversible electrophoretic deposition at sufficient electric fields and duration, which aligned with changes in surface electrode electrochemistry. Tuning potential is widely applicable to charged nanoparticle in general, as well as ionic liquids.”
Case study: Nanodiamonds dispersed in base oil + additives
A number of recent studies have examined the effect of adding nanoparticles to oil-based lubricants, commonly referred to as “nanolubricants”. Many of these studies report significant reductions in friction (typically 10%-20%) and wear, although the proposed mechanisms by which these effects are achieved vary significantly.2 Krim concurs with this and shares that friction, not wear, now dominates the financial costs of tribology
(see Figure 9); the financial costs have themselves remained nearly constant, 1.39% of GDP in 2016 versus 1.36% of GDP in 1966.
11-13 Krim says that the addition of nanodiamonds into oil can reduce friction by 75% or more, referencing studies by Ivanov and Shendarova.
2 '
Figure 9. Krim's graph of the % contributions to the financial costs of tribology broken down by category, comparing 2016 values11 (red) to 1966 values13 (black).
When nanodiamonds are mixed with a conventional zinc dithiophosphate (ZDDP) and base oil, there is a friction reduction and 3%-5% increase in fuel efficiency.
8
With the nanodiamonds experiment, Krim says that the following was observed:
14
•
The tricresyl phosphate (TCP) thermal reaction film formation temperature on iron (Fe) and 304 stainless steel (SS) surfaces is observed to be 210ºC. No film was observed to form on chromium (Cr) and air baked Fe surfaces.
•
The thermal reaction film chemical composition, as probed by energy dispersive spectroscopy (EDS), is consistent with that formed by TCP tribofilms comprised of inorganic iron phosphates.
•
Average thermal reaction film thicknesses of 70 nm and 85 nm formed over an estimated time interval of 30 minutes, corresponding to 2.3 nm/min and 2.8 nm/min respectively for the Fe and 304SS surfaces.
•
Nanodiamonds enabled the formation of reaction film on baked Fe which otherwise did not form the reaction film.
•
Nanodiamonds delayed the formation of thermal reaction film by a modest amount (18ºC), with little change to the rate of formation and the thickness of the reaction film.
•
Nanodiamonds were observed to be embedded into the film making the film stiffer. They increased the internal stress on the reaction films thereby enhancing the load bearing capacity.
•
Non-uniform distribution was observed.
In summary, Krim says that the nanodiamonds delay the reaction, but it is synergetic. For just TCP without nanodiamonds there is a reaction and bonding to the surface. When nanodiamonds are present, they get embedded into the TCP reaction and bonding, so it does react in the suspension but then still forms a film. Thus, overall they are synergistic.
14 Overall, the following was observed:
•
Film formation temperature increased by 18ºC.
•
Nanodiamond was embedded into the film, non-uniformly.
•
No change was observed in film thickness.
•
There was enhanced film adhesion and stiffness.
•
Load bearing capacity was possibly improved.
•
The abrasiveness of the film had yet to be determined.
•
Contact angle, friction and roughness were all altered.
Krim says that ZDDP and nanodiamond studies did not have as much of an effect, if any, on the reaction temperature. Overall, it nonetheless was also synergetic, as the load bearing capacity was possibly improved.
•
Nanodiamond was overall synergistic when blended with ZDDP.
•
Nanodiamond embedded into the film.
•
Nanodiamond increased the film thickness, and film adhered nanodiamond enhanced film adhesion and stiffness.
•
Load bearing capacity was possibly improved.
•
There was possible reduction in the amount of ZDDP required without compromising performance.
Outlook
Krim explains the future outlook: “First of all, nanoparticles, including nanodiamonds, have really great potential. They can contribute to energy efficiency in new and old technologies. We have been able to control them externally, and we were hoping for more uniformity and response, but everything responds differently according to the particle size, charge, etc. When we put them in oils, conventional oils alongside conventional additives were generally synergistic. For taking these quick measurements with the QCM they are correlating quite well so far with macroscale measurements in water environments.”
•
Nanodiamonds have great potential for contributing to energy efficiency and new technologies.
•
They can be controlled externally, however exhibit highly specific responses to external parameters.
•
They are generally synergistic when added to conventional lubricants.
•
QCM measurements correlate with macroscale friction coefficients in like materials.
Possible future applications include the following:
•
Active positioning of charged constituents in nanoparticle suspensions and ionic liquids.
•
Active control of nanoparticles with asymmetric charge distributions/ directed assembly.
•
Unanticipated environmental issues? Do the pros outweigh the cons? More energy to manufacture the nanoparticle than the energy reductions that they produce? Health hazards, particularly to air quality?
Summary
Nanoparticles are of increasing interest for technological applications due to their ease of production, biocompatibility and environmental friendliness, which contrasts with many current additives used in oil which are toxins.
2 Understanding mechanical and tribological properties (viscosity, friction/lubricity, wear and abrasiveness, health impact), particularly under electromagnetic fields, is vital to predicting overall device performance while using nanoparticles, both due to the reliance on nanoparticle flow in devices and since some nanoparticles can be charged in suspension.
4,8-10
REFERENCES
1.
Krim, J. (2019), “Controlling friction with external electric or magnetic fields: 25 examples,”
Frontiers in Mechanical Engineering, 5.
2.
Ivanov, M. and Shenderova, O. (2017), “Nanodiamond-based nanolubricants for motor oils,”
Current Opinion in Solid State and Materials Science, 21 (1), pp.17-24.
3.
Krim, J. (1996), "Friction at the atomic scale,"
Scientific American, 275 (4), pp. 74-80.
4.
Liu, Z., Leininger, D., Koolivand, A., Smirnov, A.I., Shendarova, O., Brenner, D.W. and Krim, J., (2015), “Tribological properties of nanodiamonds in aqueous suspensions: effect of the surface charge,”
RSC Adv., 5, 78933.
5.
Acharya, B. and Krim, J. private communication.
6.
Acharya, B., Pardue, T. N., Su, L., Smirnov, A. I., Brenner, D. W., & Krim, J. (2019), "Nanotribological performance factors for aqueous suspensions of oxide nanoparticles and their relation to macroscale lubricity,"
Lubricants, 7 (6), 49.
https://doi.org/10.3390/lubricants7060049
7.
Pardue, T. N., Acharya, B., Curtis, C. K., & Krim, J. (2018), "A tribological study of y-Fe
2O
3 nanoparticles in aqueous suspension,"
Tribology Letters, 66, 1-13.
8.
Acharya, B., Seed, C.M., Brenner, D.W., Smirnov, A.I. and Krim, J. (2019), “Tuning friction and slip at solid-nanoparticle suspension interfaces by electric fields,”
Scientific Reports, 9 (1), 18584.
Dr. Yulia Sosa is a freelance writer based in Peachtree City, Ga. You can contact her at dr.yulia.sosa@gmail.com.