HIGHLIGHTS
•
Copolyesters derived from a polyesterification reaction have been developed that show potential for removing biofilms from surfaces.
•
Adjusting a parameter known as lower critical solution temperature (LCST) is important in designing a copolyester that is able to effectively remove a biofilm.
•
At the LCST, the copolyester splits into dense and dilute phases with the former being more effective at removing biofilms from hydrophilic surfaces.
Bacteria and other microbes tend to congregate on surfaces in biofilms that are exposed to media, which is used to provide nutriment. Biofilms can be severe threats to human health and can also be a problem in water-based metalworking fluid systems where microbes can use the lubricant as a growth medium leading to fluid failure and worker health and safety issues.
Abraham Joy, professor and chair of the Bioengineering Department at Northeastern University in Boston, Mass., says, “Biofilms are organized communities of planktonic bacteria that are able to adhere to surfaces through the use of secreted biomacromolecules that include carbohydrates and proteins. They can grow extensively on surfaces even under extreme chemical and physical stresses.”
Biofilms exert a considerable economic impact that is much greater than $1 trillion annually in medical, human health and industrial applications. In the book “Metalworking Fluids, Third Edition,”
1 there is a discussion of how biofilms form and their negative effect on metalworking fluid systems. Once planktonic bacteria appear in a metalworking fluid system, mature biofilms can develop within one to two days. The biofilm can initially take components from a metalworking fluid out of the system leading to a reduction in performance that may eventually cause failure. Other negative concerns include corrosion and the generation of byproducts. The latter may result in harm to workers.
Current strategies for removing biofilms are focused mainly on using conventional antibiotics, biocides or enzymes to neutralize the bacteria within them. Joy says, “The problem is that many of the bacteria within biofilms are metabolically dormant and hence not susceptible to such approaches. These methods do not negatively impact the mechanical integrity of biofilms leaving them present, and in a position to continue to negatively affect their environment whether it be a living organism or an industrial setting such as a metalworking fluid system.”
The presence of persistor cells that can survive antibiotic treatments is thought to be one of the reasons for bacterial resistance resulting in biofilm persistence. Bacteria are protected by the biofilm matrix and can reestablish biofilm formation when necessary.
Prior to his research group moving to Northeastern University, Joy and his colleagues at the University of Akron identified that viscoelasticity and surface-adhesive properties are critical in the design of polymers that maximize surface adhesion. The researchers were inspired by the mechanisms of how marine organisms such as mussels and barnacles anchor to underwater surfaces despite the presence of biofilms in their design of synthetic equivalents of such fluids used by barnacles to clear bacterial surfaces.
Joy says, “We were inspired by work of others that demonstrated barnacle adhesion through secretion of a phase-separating fluid that is rich in lipids and phenolic compounds. These fluids are able to oxidize and remove biofilms from underwater surfaces.”
The researchers have now designed synthetic polymers that mimic this phenomenon and show promise in removing biofilms from surfaces.
Phase-separating polyesters
The key to the success of the marine organisms is that the fluid secreted exhibits phase separation behavior in water. Joy says, “During phase separation, the fluid splits into dense and dilute phases. This phenomenon is dependent upon the temperature of the environment. Our approach was to synthesize copolyesters through a polyesterification reaction between two amine-functional diol monomers and the dicarboxylic acid, succinic acid. The resulting polymers are soluble in water at low temperatures (4-10°C). Upon heating the aqueous solution, the polymer undergoes a phase separation at the lower critical solution temperature (LCST), which can be tailored by the polymer composition and, in this case, we tailor it to around 10°C.”
LCST is analogous to the term cloud point that describes the inverse solubility of some polymers in water. As the temperature of a solution increases, the polymer will eventually become insoluble turning the aqueous solution from clear to cloudy.
The researchers initially evaluated the copolyesters on biofilms grown from the bacterium,
Pseudomonas aeruginosa. Application of the dense phase of a copolyester onto the biofilm grown on simple glass channel led to the almost complete removal of the biofilm
(see Figures 1 and 2).
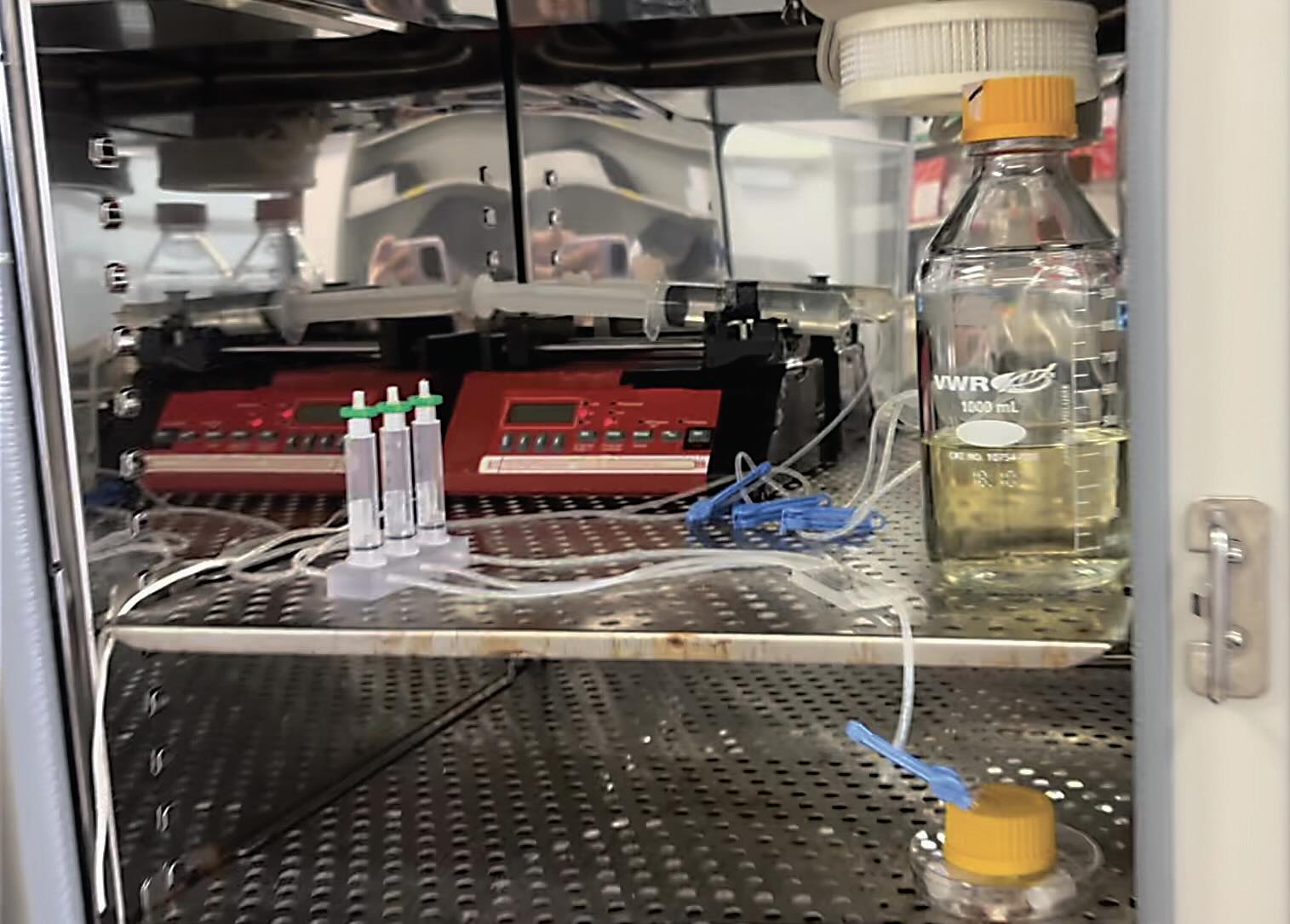
Figure 1. This experimental set-up was used to evaluate the potential for copolyesters derived from a polyesterification reaction to remove biofilms. Figure courtesy of Northeastern University.
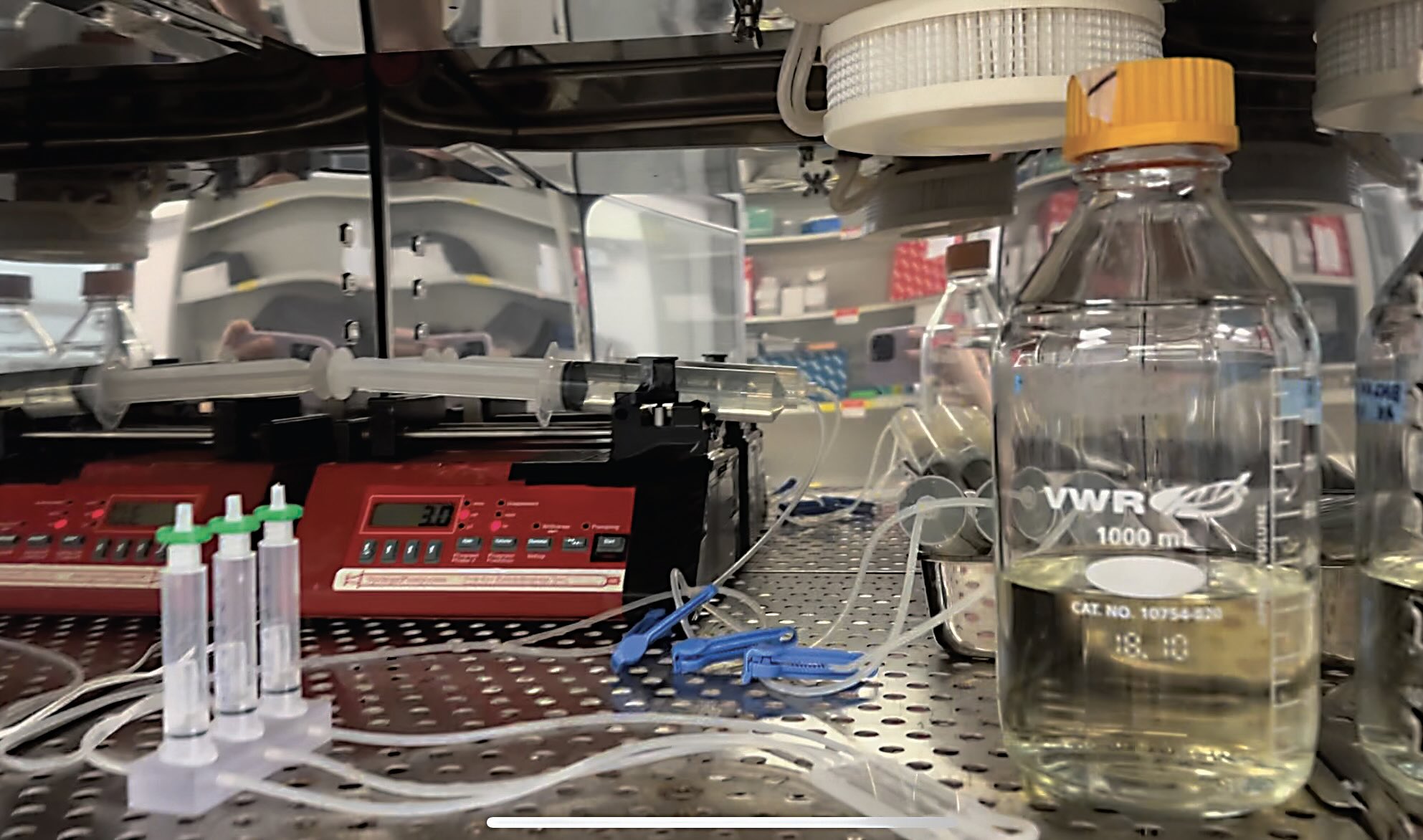
Figure 2. A second view of the experimental set-up used to assess the effectiveness of copolyesters in removing biofilms. Figure courtesy of Northeastern University.
Further testing was done on biofilms grown from other bacteria including
Klebsiella pneumoniae, Escherichia coli and
Staphylococcus aureus. Biofilm removal was somewhat effective on the first bacterium while the biofilms for the other two bacteria remained intact.
Joy says, “The mixed performance in removing biofilms is not surprising because each bacterium species establishes a biofilm with different structure, chemical composition and mechanical characteristics. The two bacteria where biofilm removal was more successful are polysaccharide-rich in composition. In contrast, the two bacteria that were unaffected are based on protein-rich matrices.”
Further testing by growing biofilms of
Pseudomonas aeruginosa on functionalized glass surfaces provided an indication of how the interfacial properties of the dense phase of the copolyester affect biofilm removal. Joy says, “We found that the dense phase is much better at removing biofilms from hydrophilic as compared to hydrophobic surfaces. This factor is an indication that the copolyester is surface active. Evidence was also found that the copolyester fractures the biofilm from the glass substrate by interfering with the adhesive bonds between the biofilm and the surface.”
Future work will consist of determining the mechanism for how the copolyester removes the biofilm produced by
Pseudomonas aeruginosa on the molecular level. Joy adds, “We will also be working to modify the copolyester to figure out how to improve its ability to remove biofilms created by other bacteria. With most biofilms containing multiple bacteria, the copolyester’s structure and surface active properties will need to be tailored to remove biofilms in specific environments.”
This unconventional approach to removing biofilms is showing promise that may eventually lead to the development of an agent that can remove biofilms situated in metalworking fluid systems. Additional information can be found in a recent article
2 or by contacting Joy at
a.joy@northeastern.edu.
REFERENCES
1.
Passman, F. (2018), “Microbiology of metalworking fluids,” in Byers, J. editor,
Metalworking Fluids, Third Edition, Taylor & Francis LLC, Boca Raton, Fla., pp. 241-283.
2.
Vishwakarama, A., Narayanan, A., Kumar, N., Chen, Z., Dang, F., Menefee, J., Dhinojwala, A. and Joy, A. (2024), “Coacervate dense phase displaces surface-established
Pseudomonas aeruginosa biofilms,”
J. Am. Chem. Soc., 146 (38), pp. 26397-26407.