Sustainably sourced electric vehicle (EV) drivetrain lubricants also can be more environmentally benign and easier to recycle or dispose of than their petroleum-based counterparts.
Evolving EV designs and fluids present a challenge to lubricant developers as well as an opportunity to distinguish themselves in the marketplace.
Biobased EV lubricants offer long-term performance and lifecycle benefits that can outweigh their higher initial cost.
If biobased lubricants are less well established and more costly than their petroleum-based and synthetic counterparts, what’s the advantage to using them? One motivation is environmental effects. Mineral oil-based lubricants are not biodegradable, and they are harmful to the environment and humans. An estimated 30-40 million tons of lubricant are consumed annually, and about 55% of this lubricant eventually enters the environment, contaminating water and soil. Because of improper disposal practices, accidental spillage and leakage, evaporation and intentional application as total-loss lubricants, over 95% of the lubricants entering the environment are petroleum based.
1
Biobased lubricants, derived from renewable resources (e.g., agricultural products and waste materials), are more environmentally benign, they are nontoxic and biodegradable, and they present fewer disposal issues than petroleum products
(see Figure 1). Plant oils, currently the most common feedstock for high-performance biobased lubricants, have good lubricity, low volatility and high viscosity indices. Many lubricant additives are soluble in plant oils, and the oils themselves are miscible in other fluids. The principal drawbacks to unmodified plant-based oils are low oxidative stability and poor low-temperature characteristics. Additives, including antioxidants and pourpoint depressants, nano-additives and emulsification, have been demonstrated to diminish those deficiencies, as have chemical modifications like transesterification, estolide formation and epoxidation. However, further research is still needed, in particular, for electric vehicle (EV) driveline applications.
2
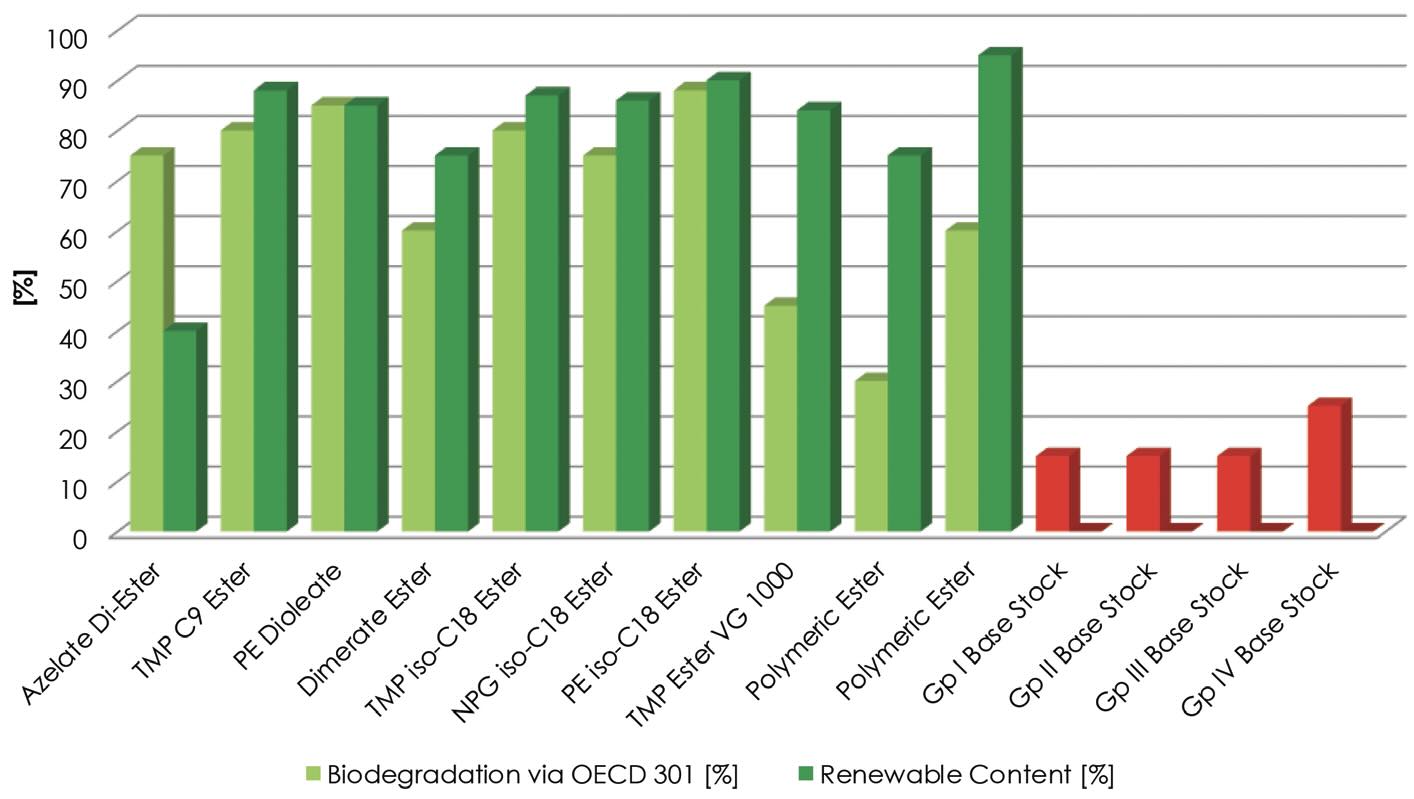
Figure 1. Renewable content (dark green) and biodegradability (light green) for various types of ester base stocks, compared with base stocks in Groups I to IV (red). Figure courtesy of Emery Oleochemicals Bio-Lubricants Division.
EVs: A market in flux
Sustainable lubricants go hand in hand with the sustainability motivation for e-mobility in general, says STLE member Dr. Matthias Hof, global business manager for BIOLUBES Ester Bio-Lubricants, Emery Oleochemicals GmbH. The expectations for sustainability and the perception of and receptiveness to “government coercion” vary in different regions of the world—the EU, U.S., China, India and elsewhere. Lubricant manufacturers must be aware of the political climate of the countries where their customers are located. “There is a perception that lubrication plays a minor role, but without lubrication in general, everything moves more slowly and does not even work at all. Etwas läuft wie geschmiert [things run much better when they are lubricated],” he adds, referring not only to the lubricants themselves, but also the regulatory drivers and other motivators for adopting them.
EV hardware is evolving quickly, and OEM targets are evolving as well. “It’s challenging to understand their expectations because of a lack of unified standards in the EV industry at this time,” Hof says. Interest in both e-mobility and sustainability expanded quickly during the COVID-19 pandemic, he adds, when there was less direct interaction between industry stakeholders and fewer people were traveling. Although he can’t say whether the pandemic influenced this interest, he notes that it will be interesting to see how things develop now that travel is experiencing a resurgence.
EVs—hybrids as well as fully electric—present a different environment and a different set of parameters than ICE vehicles, Hof says. Most EV fluids currently in use have been adapted from existing formulations, he adds. They have been tested in the field and proven reliable for their applications and extended with additional test data because of the new working environment. Development of specialized fluids designed specifically for EV applications is in the early stages, he says, with products coming to market over the next few years. Hof says, “There is still a lot of development work and innovation necessary and possible for our technology to support the development of the final driveline lubricants while the move to electromobility continues.”
One challenge to determining the necessary components in a product portfolio, Hof says, is the fact that many OEMs are unsure of what they need in the final specifications for the fluids they use, especially where EV environments differ from those found in ICE vehicles. This poses challenges in setting specific targets for fluid properties like conductivity, for example, and whether these targets should be expressed as conductivity, permittivity or resistance. Each of these properties requires a different test method. Industrial stakeholders are starting to come together, Hof says, to better understand how fluids work in automotive environments that include electric fields, new materials, faster rotational speeds and different loads and temperature regimes. How can base oils, additives and esters that can serve both functions influence performance in this new environment?
This is the biggest change that has come to the lubricant industry in years, Hof says. Currently, he adds, esters are still considered to be somewhat of a niche product that demand a price premium. Lately, however, lubricant formulators are showing a greater interest in esters because their stability in electric fields, their friction and wear prevention properties and sustainability benefits are seen as justifying their higher cost.
Jason Rosalli, formerly vice president of research and development at Novvi, LLC, notes that each EV design is different, and each OEM is looking to achieve different things. Thus, fluid suppliers must deliver products that meet specific OEM requirements, rather than relying on the standard formulations typical of ICE vehicle fluids.
Even without the vehicle design differences, high-speed EV motors create new challenges, including foaming and aeration, he says.
3 Other challenges include understanding where the viscosity “floor” is for EV driveline fluids—how low can these viscosities go and still be effective? Immersion cooling for gears and drivetrains also is an active area of research. “Every base stock and every additive is going to have a different role to play there. It’s something that everyone will need to develop and our material, like everything else, will have a slightly different effect. Even PAOs from different suppliers may have different effects,” he says.
However, for some lubricant companies, business considerations necessitate that biobased EV drivetrain lubricants remain on the horizon. Chet McBroom, vice president of commercial sales, West Coast, for Renewable Lubricants, Inc., notes that he doesn’t currently have any active research on biobased EV lubricants. Most of the current demand for EV lubricants comes from OEMs who want factory fill fluids with at least a 10-year lifespan, he says. Thus, aftermarket demand is likely to be limited for the next decade or so. “Unless we have an OEM interested in partnering with us, it’s not likely we will be in this market anytime in the near future,” he says.
Rosalli, on the other hand, has received feedback from various parties indicating interest in adopting novel fluids before the EV fluids in vehicles on the road today will need replacing. There is a challenge but also an opportunity for novel fluids that meet specific OEM issues or requirements, he says—more so than in ICE oils, where there are distinct grades and everyone follows API guidelines. “This is actually an opportunity to derive more value and to differentiate yourself from other suppliers,” he says.
Larger companies with major research and development programs also are working to stay ahead of the curve on sustainability. STLE member Mike Barry, strategic marketing manager, Fuel and Lubricant Solutions, for BASF, notes that his company, like many others, is “working on finished lubricant formulations that meet the unique requirements of electric drive components and will complement the existing portfolio of axle and transmission lubricants.”
Feedstocks from many sources
Although animal fats have been studied as additions or replacements for petroleum-based lubricants, plant-based oils are emerging as the most promising candidates.
2 For automotive applications, the focus is on non-edible oil seed crops including shrubs and trees, some of which are already used for medicines, personal care products and lubricant base oils for other applications. Microalgae and other microbial sources, an emerging third-generation source of biobased lubricants, are an active area of research.
The balance of advantages and challenges in the choice of a feedstock is complex. Despite their many advantages, high production costs currently limit plant-based biolubricants to about 1% of the total lubricant market. Some 75%-80% of production costs are determined by the cost of plant-based feedstocks, driven by the time and energy required to cultivate and harvest oil-producing crops.
1 Diverting edible crops for use as lubricants could have detrimental effects on food supplies, and even inedible agricultural products require land space that could be used to grow edible crops.
Animal fats could be a less expensive, more sustainable alternative.
1 Raising livestock requires significant resources in terms of water, crops and grazing land, but animal fats are a byproduct of existing meat processing and rendering operations. As long as people continue to eat meat, there will be a reliable supply of animal fats from these operations. Health concerns prevent much of the animal fat produced in the U.S. from being considered edible, so using this fat for biobased lubricants will not compete with food supplies.
Rosalli says that feedstocks that are plant-based oleochemicals, rather than crude plant oils, must fit the same exacting specifications, whether the source is coconut, palm, soy or some other source. Some companies convert these feedstocks into saturated hydrocarbons, including hydroisomerized, branched hydrocarbons. By optimizing the branching of the hydrocarbon molecules, the desired physical properties are achieved. “The idea is not to make a me-too product that replicates something else, it’s to make a different optimization that will give you beneficial efficiency gains in the application,” he says.
Manufacturers of ester products also must modify their feedstocks to meet the high-performance requirements of EVs. Natural or naturally derived esters are derived from plant oils and fats (palm, coconut, soy and others) or from animal sources like tallow, lard or chicken fat. They are sometimes used by the industry for moderately demanding applications like in metalworking fluids and chainsaw oils, for example, where they function as “lost lubricants” rather than in fill-for-life or other long-life applications, Hof says.
Hof explains that synthetic esters are better suited to the performance requirements of EV motors and drivetrains. Group V oils, which include esters (e.g., estolides derived from renewable resources
4), can be made from various feedstocks, but EV lubricant components must meet the performance standards required by high rotational engine speeds and temperatures, materials compatibility, along with performance stability in an electrical field.
Ester manufacturers can combine specific acids and alcohols to customize their products to meet specific performance requirements, Hof explains
(see Figure 2). The acids and alcohols can come from petrochemical sources, but the acid part also can be sourced from renewable origins like naturally occurring triglycerides. However, acids derived from natural products always contain molecules spanning a range of carbon chain lengths and degrees of saturation. For example, tallow contains molecules in the C16 to C20 range, sunflower oil spans the C14 to C20 range and coconut oil has molecules in the C8 to C14 range. The range of carbon chain lengths and degrees of saturation in any particular plant source (even different varieties of the same plant) has an effect on the properties of the final product. But this variability is not necessarily a problem, Hof explains, noting that ester producers can use these various acid characteristics to fine-tune the viscosity profile, low-temperature profile, oxidative stability, volatility, intrinsic lubrication and solvency of the ester, which can then be used in the finished lubricant.
Figure 2. Ester manufacturers can customize their products to meet specific performance requirements: traction coefficients, for example. Traction coefficient versus slide-to-roll ratio (SRR) for various esters compared to PAO-2 (top) and blends of PAO-2 with various esters (bottom). Figure courtesy of Emery Oleochemicals Bio-Lubricants Division.
Where do animal-derived fatty acids come into play? One of the most commonly used oleochemical esters in the industry, Hof says, is trimethylolpropane trioleate (TMPTO), made from trimethylol propane and oleic acid
(see Figure 3). “If you buy TMPTO made in the U.S., I guarantee that it is based on animal fatty acids,” Hof says. Plant fatty acids predominate in Asia and Europe, and they are becoming more widely used in the U.S., he adds, but tallow has been the main source of oleochemical feedstocks in North America since the 1990s. “Meat consumption in the U.S. is among the highest worldwide,” he says.
Figure 3. Trimethylolpropane trioleate (TMPTO) is one of the most commonly used oleochemical esters in the industry. Figure courtesy of Emery Oleochemicals Bio-Lubricants Division.
Hof notes that some ester products currently in use are based on highly fractionated fatty acids. However, because of the composition of the fatty acid used, the industrial TMPTO ester contains only about 70%-75% oleic acid, with the rest being a mixture of palmitic, stearic and linoleic acids. Purifying the raw material further would drive up the cost. Hof adds that finer fractionations to separate out the various acids might be necessary as formulation needs become more specialized.
Tallow also is a sustainable feedstock for energy production, which has driven a recent price hike. Four years ago, tallow costs were running about 30 to 35 cents per pound, Hof says. Since then, the price peaked at about 75 cents per pound, and it is currently running about 50 to 55 cents per pound. This has opened an opportunity for Southeast Asian producers of palm, palm kernel oil and corresponding fatty acids to export their products to the U.S.
Some industry sectors, like cosmetics and personal care products, have moved away from animal-based oleochemicals for marketing purposes, although there is no significant chemical difference between a given oleochemical compound derived from plants and one derived from animal sources. Other sectors are staying with their animal-based oleochemicals to avoid the effort and expense of reapproving their products using plant-based materials. In the EU, new deforestation regulations are coming into effect in 2024 and 2025, and this adds an additional complication. “The oleochemical market is currently very dynamic,” Hof says.
Microbial products like alginates could provide a source of oleochemicals that sidesteps the problems associated with meat and crop production, but they are still a high-cost niche product, Hof says. This could change depending on what happens with more mainstream sources of oleochemicals and technological developments in microbial and biosynthetic processes. Some companies also have been looking into oleochemicals from waste streams like food scraps, and used cooking oil has been used as a raw material for several years. However, chemicals from these sources must be refined and derivatized to meet the standards necessary for high-performance applications like EV lubricants.
Recycled base oils, so-called re-refined oils, are improving in quality, Hof says, and they are reaching the point where they could be used for more advanced applications. However, these oils are usually based on petrochemicals. “The renewability and recyclability aspects are one piece of the sustainability puzzle,” Hof says. They are an important piece, but not the whole picture, he adds. “If a product is environmentally benign but so expensive that no one can afford to use it, it will not work.” Another aspect is factors involved in obtaining the raw materials—deforestation to make room for the oilseed crops used to make oleochemicals, for example. “Does the way to get there negate the benefits?” Hof asks. Perhaps—a 1% or 2% gain in vehicle energy efficiency for an individual vehicle, multiplied over millions of vehicles adds up to a significant effect overall, he adds.
Properties for performance
Regardless of the source, EV lubricants and their components must be up to the task of performing in a challenging environment. In addition to having low viscosity and good electrical properties, EV transmission oils must perform well under sustained operating speeds exceeding 20,000 rpm and be compatible with copper wiring, insulating materials, seals and electrical contacts.
5 Biobased lubricants check all these boxes, as well as offering low compressibility, efficient air release properties and high flash points. On the other hand, the underivatized versions of these oils tend to be oxidatively unstable, and high concentrations of additives and thickening agents can cause abrasive wear problems.
Biobased oils are mostly unsaturated fatty acids; that is, they contain reactive carbon-carbon double bonds that keep them liquid at room temperature, but also make them susceptible to oxidation or thermal degradation. Various methods of converting biobased oils to saturated fatty acids (e.g., esterification, epoxidation, hydroxylation, isomerization or isopropylation) make them more stable with respect to oxidation, but they also affect other tribological properties like viscosity, and chemical modification can be used to give these oils better cold-flow properties.
Esters have been used in automotive formulations and driveline fluids for a long time because of their lubricity, seal compatibility and solvency. Solvency is a particular concern, Hof says, because as high-quality base stocks are increasingly refined—Group II, III and Group IV base stocks have essentially no sulfur—they become less polar. Ester cosolvents can provide the polarity needed to dissolve additives, stabilize the formulation and provide cleaner operations through the uptake of degradation products.
Other polar additives include certain bio-ionic compounds, formed as salts of biopolymer cations, which outperform commercial PAOs in friction and wear protection and adhere well to metal surfaces. For example, tert-butylammonium derivatives of amino acids outperform halogen-containing ionic lubricants in protecting against corrosion and resisting hydrolysis, and they are less costly to produce.
5
On the nonpolar end of the spectrum, saturated hydrocarbons have no oxygen content, so they are as oxidatively stable or slightly more stable than PAOs, says Rosalli. Some hydrocarbons have a lower coefficient of friction than PAO oils, very similar to the metallocene-based low-viscosity lubricants coming out for EV drivelines. Plant-based hydrocarbon base oils can be blended with metallocene oils to achieve an optimal viscosity for the application. “We are seeing a market trend going toward lower viscosity and a lower coefficient of friction,” Rosalli says.
Various types of plant-based oils have been tried as lubricants, but not all oils are created equal. For example, castor oil has been used for many years as an engine lubricant for race cars, diesel engines and jet engines because it performs well over a wider temperature range than petroleum-based lubricants. On the other hand, waste oils, such as used cooking oils or plant lubricant byproducts, lack additive technologies that can bring their performance up to the desired standards, making them impractical for use as automotive drivetrain lubricants. And certain palm oil esters can actually worsen wear on steel surfaces during boundary lubrication.
5
As base oils, plant oils offer excellent viscosity indexes, very low volatility and very high biodegradability.
1 However, plant oils are triglycerides with three fatty acids esterified to a glycerol backbone, which makes them susceptible to hydrolysis. Some oils (palm, coconut) are high in saturated long-chain fatty acids, which causes them to have poor cold-flow properties. Most plant oils (soybean, corn, sunflower) contain high percentages of unsaturated fatty acids, resulting in poor oxidative stability. However, the physicochemical and tribological properties of plant oils can be improved through chemical modifications or using additives to the lubrication formulation. The stability of plant oils can be improved by completely or partly reacting the unsaturated acids, by modifying the triglyceride structure through transesterification reactions, or by including stabilizing additives in the biolubricant formulations.
Dialkyl phosphonate derivatives of plant oils (e.g., soybean oil or high-oleic sunflower oil) form protective films during lubrication, which reduces friction-induced wear on solid surfaces. Four-ball tribometer tests have shown that these biobased phosphonate additives provide similar or better friction properties than the commercial petroleum-based phosphate additives commonly used in motor oils. Plant oil phosphonate derivatives can be poorly soluble in PAO-6, but most of them have adequate solubility in high-oleic sunflower oil. When these phosphonates are added to a high-oleic sunflower base oil blend, they demonstrate similar coefficients of friction and similar or better wear scar diameters than the common inorganic antiwear agent zinc dialkyldithiophosphate (ZDDP).
6
Various additive schemes have been investigated in the context of synergy between antioxidants and antiwear additives for use with plant oils.
7 Dialkyldithiocarbamate antioxidants have been shown to outperform the diphenylamine or hindered phenolic additives typically used with mineral oils. Zinc dialkyldithiocarbamate antioxidants perform synergistically with antimony dithiocarbamate antiwear additives.
In comparison with plant oils, animal fats contain significant amounts of saturated fatty acids, along with proteins and phospholipids.1 Chicken fat, generated during chicken feather rendering, contains triglycerides composed of about 32% saturated and 65% unsaturated fatty acids, including palmitic, stearic, oleic and linoleic. Converting the double bonds in the unsaturated fatty acids to isopropyl branches improves the oxidative stability of the fats, as well as increasing the viscosity and density.
Isopropyl-modified chicken fat is denser than unmodified chicken fat, but it shows similar solubility in high-oleic sunflower oil and in PAO-6.1 The modified chicken fat has better oxidative stability, higher kinematic viscosity and a lower viscosity index than either unmodified chicken fat or high-oleic sunflower oil. Blending modified chicken fat with high-oleic sunflower oil increases its density and oxidative stability—unmodified chicken fat does not provide these benefits when blended with high-oleic sunflower oil.
Beef tallow, composed of unsaturated fatty acids, is another animal-based alternative or supplement to plant-based formulations.
8 Alkylating beef tallow’s double bonds with isopropyl groups completely saturates the fatty acids, and this branched beef tallow (BBT) is more stable toward oxidation. Unmodified beef tallow and BBT both have a lubricity similar to high-oleic sunflower oil, and adding as little as 5 wt% of either of these forms of tallow improves the lubricity of PAO-6. The isopropyl branching and saturation in BBT improve its solubility in high-oleic sunflower oil and PAO-6, and they lower its viscosity with respect to unmodified beef tallow. The saturation also means that BBT has better low-temperature properties than unmodified beef tallow, both in its neat form and as a blend with high-oleic sunflower oil or PAO-6.
In comparison with commercial petroleum oils with antiwear additives, chemically modified plant oils often come up short. However, several types of binary metal oxides or chalcogenide particles have been used effectively as base oil-compatible wear prevention additives in modified plant oils.
9 One study compared coconut oil (more than 90% saturated fatty acids) and mineral oil (500 N base oil) containing various concentrations of MoS
2 nanoparticles.
10 Particles with and without surfactant modification (for stabilizing the nanoparticle suspensions) also were compared. Pin-on-disc and four-ball tests using a sliding interface between aluminum and steel in the boundary and thin film-lubrication regimes at 30°C and 120°C showed that the nanoparticles reduced both friction and wear in comparison with the base lubricants alone.
The ability of nanoparticles to improve antiwear and extreme pressure (EP) performance also was demonstrated in a study comparing 1 wt% MoS
2 or 1 wt% CuO nanoparticles added to chemically modified palm oil.
9 Treating the nanoparticles with 1% oleic acid as a surfactant stabilized the suspension and reduced sediment formation. The study showed that the MoS
2 nanoparticles reduced wear better and had better EP performance than did the CuO nanoparticles. This finding supported the results of a previous study showing that adding CuO and ZnO to biolubricants based on sunflower and soybean oils did not effectively enhance wear protection.
Worth the expense?
Does the performance of sustainable lubricant components justify the higher cost of feedstock sourcing and formulation development? If you’re going strictly on a dollars-per-pound comparison, Hof says, then nine times out of 10, the petrochemicals will be less expensive. On the other hand, comparing products on a cost-for-performance basis (measured with bench testing or rig testing) shows benefits in reducing friction, improving wear protection through intrinsic lubrication and high metal surface adsorption. Thus, the benefits go to the customer rather than the fluid formulator, Hof says, in the form of longer vehicle life, less down time for maintenance and repairs, better energy efficiency and lower disposal or recycling costs.
Determining costs and benefits across an entire lifecycle analysis is complex, Hof says, and it involves setting priorities and determining what trade-offs are acceptable.
11 “If I have a toxic compound that offers significant improvements in energy efficiency, is that acceptable? What is the balance in the long run?” he asks, hypothetically. In the past, customers might have prioritized equipment protection over environmental factors or energy efficiency. As energy has become more expensive and environmental issues have taken on greater urgency, he says, this balance has shifted.
Although the initial cost of these products may be higher than for conventional oils, Rosalli says, the improved efficiency and sustainability should pay off over the life of the fluid. He notes that efficiency gains also reduce a product’s in-use carbon footprint by reducing the amount of energy needed to do the same amount of work. The best gains show up in a cradle-to-grave analysis, Rosalli says, citing API Technical Note 1533, which he helped to author.
12
Plant-based hydrocarbons are easier to recycle or re-refine because the hydrocarbons can be dropped directly into the refinement stream, says STLE member William Downey, Novvi’s senior vice president of business development.
Barry notes that because biobased lubricants combine sustainability benefits while maintaining performance integrity, these products can offer a strong value proposition. “Cooperation across the supply chain is essential to understand the value of biobased lubricants,” he says. He adds, “For biobased finished lubricants, it is important to build performance confidence, assist customers with lifecycle assessment calculations and ensure sufficient supply chain capacity to meet demand.”
REFERENCES
1.
Yosief, H. O., Sarker, M. I., Bantchev, G. B., Dunn, R. O. and Cermak, S. C. (May 2022), “Physico-chemical and tribological properties of isopropyl branched chicken fat,”
Fuel, 316, doi: 10.1016/j.fuel.2022.123293.
2.
Farfán-Cabrera, L. I. (2019), “Tribology of electric vehicles: A review of critical components, current state and future improvement trends,”
Tribology International, 138, pp. 473-486,
https://doi.org/10.1016/j.triboint.2019.06.029.
3.
McGuire, N. (2024), “Bubble trouble in electric vehicle fluids,” TLT,
80 (7), pp. 62-72. Available at
www.stle.org/files/TLTArchives/2024/07_July/Feature.aspx.
4.
Canter, N. (2024), “Sustainable synthetic lubricant base stocks: Significant influence in the move to sustainability,” TLT,
78 (12), pp. 38-48. Available at
www.stle.org/files/TLTArchives/2022/12_December/Cover_Story.aspx.
5.
Bradu, C. I. and Ianus, G. (2022), “A prospective view on biodegradable lubricants for hybrid and electric vehicles,” IOP Conference Series: Materials Science and Engineering, 1262 (2022) 012001, doi: 10.1088/1757-899X/1262/1/012001.
6.
Biresaw, G., Bantchev, G. B. and Harry-O’Kuru, R. E. (Jan. 2021), “Phosphonates of vegetable oils—Characterization as lubricants,”
JAOCS, Journal of the American Oil Chemists’ Society, 98 (1), pp. 89-102, doi: 10.1002/aocs.12448.
7.
Sharma, B. K., Perez, J. M. and Erhan, S. Z. (July 2007), “Soybean oil-based lubricants: A search for synergistic antioxidants,”
Energy and Fuels, 21 (4), pp. 2408–2414, doi: 10.1021/ef0605854.
8.
Yosief, H. O., Sarker, M. I., Bantchev, G. B., Dunn, R. O. and Cermak, S. C. (July 2022), “Chemical modification of beef tallow for lubricant application,”
Ind Eng Chem Res, 61 (27), pp. 9889-9900, doi: 10.1021/acs.iecr.2c01207.
9.
Gulzar, M., et al., (Aug. 2015), “Improving the AW/EP ability of chemically modified palm oil by adding CuO and MoS2 nanoparticles,”
Tribology International, 88, pp. 271-279, doi: 10.1016/j.triboint.2015.03.035.
10.
Koshy, C. P., Rajendrakumar, P. K. and Thottackkad, M. V. (June 2015), “Evaluation of the tribological and thermo-physical properties of coconut oil added with MoS2 nanoparticles at elevated temperatures,”
Wear, 330–331, pp. 288-308, doi: 10.1016/j.wear.2014.12.044.
11.
McGuire, N. (2023), “Sustainability in the lubricants value chain,” TLT,
79 (12), pp. 34-40. Available at
www.stle.org/files/TLTArchives/2023/12_December/Webinar.aspx.
12.
“Lubricants life cycle assessment and carbon footprinting—Methodology and best practice,” API Technical Report 1533, first edition, May 2023. Available
here.
Nancy McGuire is a freelance writer based in Albuquerque, N.M. You can contact her at nmcguire@wordchemist.com.