Meet the Presenter
Scratch the surface of invisible defects in glassy materials with this article, based on the webinar titled Tackling the Puzzle Invented by the Devil: Tribology of Glass Materials. Hosted by the American Society of Mechanical Engineers’ (ASME) Tribology Division and presented by STLE member Dr. Seong H. Kim on April 26, 2023, the session explored laboratory methods for observing defects on and just below glass surfaces, the causes of these defects and how defects affect glass performance. Courtesy of STLE, this article captures the core insights from this ASME-organized event. For more technical content from the ASME Tribology Division Webinar Series, visit the ASME Tribology Division’s website here.
Seong H. Kim is a distinguished professor of chemical engineering, a professor of materials science and engineering and a professor of chemistry at Pennsylvania State University. He works in the university’s Materials Research Institute. You can reach him at shk10@psu.edu.
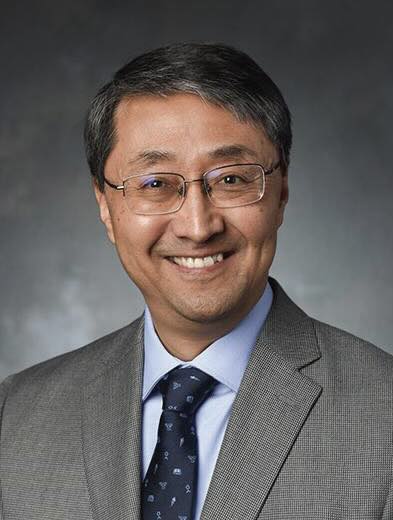
Seong H. Kim
KEY CONCEPTS
•
Invisible glass surface defects and damage can weaken glass and accelerate failure.
•
Adsorbed liquids and solid particles significantly affect how susceptible glass is to wear.
•
Physical contact produces persistent subsurface structural changes, even in regions outside the contact area.
Touch screens are a fact of everyday life; we enter our personal identification number (PIN) at a grocery checkout or ATM, or we write on our phone or tablet screens with a stylus. No harm done, right? It’s common knowledge that a harder material will scratch the surface of a softer material, but has anyone really looked at what happens at or below a glass surface when it is tapped or rubbed? How much does the glass recover from applied stress, and what effects does cumulative damage produce? How much does this damage affect the chemical and mechanical durability of the glass? Does it make a difference if the surface is wet or dry, hot or cold, surrounded by air or organic vapor?
This article is based on a webinar presented by STLE member Seong H. Kim, distinguished professor of chemical engineering, a professor of materials science and engineering and professor of chem istry at Pennsylvania State University, and the Tribology Division of the American Society of Mechanical Engineers (ASME) titled Tackling the Puzzle Invented by the Devil: Tribology of Glass Materials. See Meet the Presenter for more information.
In theory, glass should be a very strong material. “The dream is to achieve the theoretical strength limit, which is similar to hardened steel,” says Kim. However, unlike steel, glass has no grain boundaries, so surface cracks can propagate unimpeded through the material until the glass breaks. Even the advanced glass formulations used for electronic device screens, dental implants and fiber-optic cables lose strength over time, starting from the moment the glass is transported out of the factory.
1 “We always blame surface defects,” Kim says, “but we can’t see the defects below the surface.”
Glass is strong, until it’s not
Using a technique called sum frequency generation (SFG) spectroscopy, which characterizes surfaces and interfaces using overlapping infrared (IR) and visible light beams, Kim’s group found that glass with surface defects is susceptible to chemical attack in a way that defect-free glass is not. They also used microscopy to show that glass that has been rubbed is prone to chemical corrosion and dissolution by alkaline solutions.
1
Corrosion is especially concerning for glass applications like nuclear waste storage, where radioactive materials are incorporated into a glass matrix. This glassy material is stored in stainless steel containers, which are placed into concrete bunkers. However, “the ground is a hostile environment,” Kim says. Not only do sediments and rock layers shift and settle, but salty groundwater can dissolve or etch defects into glass over long periods. Groundwater entering a storage chamber through cracks in the concrete can corrode the steel containers and eventually degrade the glass matrix.
Because glass erosion is so slow, it is difficult to study in a lab setting without using accelerated aging methods. Kim cites examples of glass corrosion studies done using crushed glass. Smaller glass particles have more surface area, which accelerates the corrosion process and enables laboratory observations within a practical time period. However, the crushing process produces defects that are not present in the original bulk glass, and thus, this method does not offer a reliable simulation of a larger body of glass.
Why does this matter? Unlike metals, ceramics and other crystalline materials, which have grain boundaries that can arrest crack propagation, glass is a single-domain material. Once a crack initiates, there is nothing to stop it from growing until it fractures the glass. Various treatments can retard crack initiation. For example, potassium-doped sodium aluminosilicate phone screen glass is an example of adding small amounts of a heavier chemical element to a glass formulation. These larger atoms place the atomic network under compression, which retards crack formation. However, when a crack forms in these materials, it propagates quickly throughout the solid. Tempered glass of the type used in car windshields relies on fast cooling to produce a surface layer that is under compressive stress, with the interior under tension. Tempered glass is stronger than annealed glass, but when it does break, it shatters into thousands of small, rounded chunks, rather than large sharp-edged shards.
Older glass is easier to break, Kim says. If you press the glass surface with a small hard sphere until it indents and then remove the sphere about 8 micrometers deep, Kim says, you get about 99.97% recovery afterward, but an indentation about 3 nanometers deep remains. Is that significant? Kim’s group tried pressing a glass surface until it broke, which required about 5-6 gigapascals of contact stress. Indenting the surface a second time required less stress to break the glass, and a third indentation required still less stress. “So we’re accumulating defects,” he says.
Friction, wear and corrosion testing
Kim’s group is working to understand the behavior of glass under stress. It’s a challenge because, unlike crystalline materials, glass is in a nonequilibrium state. Its properties depend not only on its chemical composition, but also on how it has been processed— cooling rate, forming process, polishing and annealing, to name a few. They found, for example, that mechanically polished flat glass surfaces have a higher concentration of defects than fire-polished glass.
Over a period of many years, glass relaxes and releases embedded strains. Unlike crystalline materials, in which even very small strains and defects show up as changes in a diffraction pattern or polarized light micrograph, finding strained regions in glassy materials is much more challenging. Because glass is an insulator, characterization methods based on electrons and ions are not as effective as they would be for metals or semiconductors. Also, transparent glass transmits light largely unchanged, which poses difficulties for light scattering and absorption measurements.
To get a better idea of how defects and residual damage affect the strength and performance of glass, Kim’s research group is using standard tribological test methods. They are identifying and quantifying what happens at and below the surface when various types of glass meet metals, silicon nitride or other types of glass. Under a dry atmosphere, a steel, silicon nitride or alumina ball will scratch a softer soda lime silica (SLS) glass surface, with little to no damage to the ball. When water vapor is present, however, chemical reactions occur at the surface, significantly increasing wear on the ball’s surface, even though this surface is harder than the glass.
2 The wear tracks on the glass depend on the amount of relative humidity and the type of glass used.
3, 4, 5 They attribute this wear to shear-induced mechanochemical reactions involving adsorbed water molecules. The pH of the rinsing solution makes a huge difference on the water adsorption and desorption behavior on the glass surface by affecting the distribution of electrostatic charges.
Molecular dynamics simulations, in collaboration with Adri van Duin (Pennsylvania State University), showed that the severe glass surface wear in dry conditions is due to the formation of surface bonds between silicon in the substrate, interfacial oxygen and silicon in the countersurface that convey the interfacial shear stress to the subsurface. These simulations show that the presence of interfacial water reduces the formation of these interfacial bridging bonds.
6
Glass surfaces are more likely to scratch if they are dusty when someone swipes right on their phone screen or uses a cloth to clean their eyeglasses. Particle adhesion also is affected by humidity, but how humidity affects adhesion depends on the size of the particle. Microscale cellulose particles adhere more strongly to a glass substrate under dry conditions, where van der Waals forces produce adhesion, or under high-humidity conditions, where surface tension produces adhesion. In contrast, nanoscale cellulose particles adhere most strongly under intermediate humidity conditions, where Laplace pressure with a high filling angle predominates.
7
Can good lubrication protect a glass surface from damage during frictional sliding? Kim’s group tested a Pyrex glass ball on a soda lime float glass substrate, applying a 0.2 N load. The glass substrate had an adsorbed layer of alcohol, applied using vapor deposition. After 400 sliding cycles, no visual wear was observed on either the substrate or the ball.
4 However, after subjecting the flat glass plate to hot steam for 24 hours at 150°C, surface profilometry and optical imaging revealed a distinct wear track and scratches extending across this track at various angles, indicating weakened regions, even in the absence of visible wear.
8
To investigate further, Kim’s group took atomic force microscopy (AFM) topography images of samples of International Simple Glass (ISG), a six-component borosilicate glass that is commonly used in laboratory testing to facilitate comparisons and reproducibility.
9 They compared AFM images of polished samples with polished-then-annealed samples in their initial states. Then, they subjected some of these samples to mildly corrosive conditions (aqueous solution, pH 7, saturated with soluble silica, 21 days at 30°C). Other samples were subjected to harsher conditions (aqueous solution, pH 7, saturated with soluble silica, seven days at 90°C).
All of the samples showed surface scratches, but these became more pronounced in the mild-corrosion samples and even more pronounced in harsh-corrosion AFM images for the polished-only samples. For samples that had been annealed after polishing, only slight differences were observed under mildly corrosive conditions, with scratches becoming denser under the harsh conditions. Specular reflectance infrared (SR-IR) spectroscopy showed significant water ingress into polished-only glass samples (mild corrosion) but not the polished and annealed samples.
What’s going on?
What happens to a glass surface when it is physically touched? Why does the physically contacted region become more susceptible to hydrolysis? Kim’s group and their collaborators looked for laboratory tests that they could perform that would show them what happens to a glass surface—and below—on an atomic scale. They were especially interested in why a physically contacted region becomes more susceptible to hydrolysis.
Unlike crystalline materials that produce clearly defined patterns of diffraction spots, glass, with its random arrangement of structural units (SiO
4 tetrahedra, for example), yields a series of broad X-ray or neutron scattering peaks, indicative of radial distribution functions within the solid. Still, these broad peaks provide valuable information on glass network structures. Nuclear magnetic resonance (NMR) provides further information on short-range structure.
Vibrational spectroscopy techniques could be an additional source of information on damage-induced structural changes. However, the underlying assumptions and theories used to assign peaks in the spectrum are based on small, isolated molecules, Kim says. Spectroscopic interpretation cannot be done by simply comparing the detected band frequencies with those reported in the literature. Applying molecular principles to glass samples produces erroneous results because glass is a three-dimensional network of connected atoms, each of which affects the others. What’s more, glass has a large absorption cross section, so the Beer–Lambert law, which works well for liquid solutions, produces incorrect structure models for glass samples.
Various types of IR spectroscopy provide spectra using different sample configurations, and the complexity of interpreting the results can vary widely.
10 The most basic method, transmission IR spectroscopy, is fairly simple. Photons go in one side of the sample, they interact with the atoms or molecules in the sample, and they come out the other side. Attenuation total reflectance (ATR-IR) and specular reflectance (SR-IR) cannot be interpreted in a manner similar to transmission IR. The strength of the reflected beam in ATR-IR is affected by the evanescent wave it produces in the reflecting surface. For SR-IR, some of the incident beam reflects from the top surface of the glass. Some of the beam is refracted as it travels through the glass, reflects from the bottom surface, and refracts again as it exits the top surface. The remainder of the beam passes through the bottom surface, refracting as it enters and exits the glass.
Raman spectroscopy is still more complex. This technique provides structural information using frequency differences between the incident photons and the scattered photons, caused by the light beam’s interaction with the atoms in the glass. However, not all of the parameters are well characterized. Glass surfaces are more likely to scratch if they are dusty when someone swipes right on their phone screen or uses a cloth to clean their eyeglasses.
To overcome the limitations in interpreting vibrational spectra from glass, Kim’s group has been examining vibrational spectra for flat plates of silica and silicate glass. They have developed theories and structural interpretations for these materials that agree well with their observations using other methods.
11 For example, photothermal AFM-IR enables chemical identification, and the emitted IR spectrum bears a direct correlation to Fourier transform IR (FT-IR) data, without the use of models. Scattering-scanning nearfield optical microscopy (s-SNOM) nano-IR relies on nano-optical phenomena, enabling sub-diffraction- limit optical imaging.
Vibrational spectroscopy as a structural analysis tool for glass
We now have a better understanding of how to interpret vibrational spectral features of glass surfaces, Kim says. For damaged surfaces, he continues, the physical contact always occurs locally, so we need a spectroscopic imaging technique that has sufficient spatial resolution to probe the “touched” region.
Kim’s group collaborated with Slava Rotkin (Pennsylvania State University) to examine nearfield scattering 20-40 nm below a glass surface using s-SNOM nano- IR.
12 They made a nanoindentation in silica glass (Berkovich tip, 5 mN) and found that under plastic deformation, there is a decrease in Si–O–Si bond angle, indicating densification, while s-SNOM-IR showed an elongation of the Si–O bond length. Even in the topographically “elastic” region, s-SNOM-IR showed a slight elongation of this bond length. Thus, even in the elastic contact region, where the topography has fully recovered after indentation, there could be chemical plasticity—persistent subsurface structural changes in the glass.
To find out whether the “topographically elastic” edge region of the nanoindentation had been affected by the plastic deformation of the center region, the group performed Hertzian indentation of SLS glass with a 2-mm tungsten carbide sphere. They encased their setup in an environmentally controlled enclosure and mounted the sphere on the tip of an indenter equipped with a force sensor and an acoustic sensor.
13
Kim’s group performed photothermal AFM-IR studies on float glass and found that the response was nearly elastic at 3.5 GPa pressure. A ring crack began to form at 5.0 GPa, and a cone crack formed at 6.2 GPa. They established the depth below the surface at which the IR intensity decays, and they compared their AFM-IR spectra with simulations and SR-IR spectra. In collaboration with Ali Borhan (Pennsylvania State University), they simulated transient temperature profile of the glass surface upon absorption of IR and compared it with the thermal expansion profile measured with AFM-IR. Based on the agreement, they were able to interpret the AFM-IR spectra properly and found that their experimental data indeed support the hypothesis that the indentation increases the Si–O bond lengths.
14
They also observed that the direct contact region exhibited the largest change in the Si–O stretch mode, even after the surface topography had recovered by 99.97% after “nearly elastic” contact, and that this change was due to residual compressive stress. Even in the region more than 200 micrometers away from the contact center, a region that was not touched by the probe, residual tensile stress altered the Si–O stretch mode of the glass network.
13
Further, the physically contacted region became more vulnerable to chemical attack, mostly due to interfacial friction rather than compressive stress. When they slid the indenter tip across the surface, the interfacial friction caused the topmost region to become more susceptible to base-catalyzed hydrolysis, even when the load was much lower than the nominal yield strength of the glass.
13
Invisible, but real, damage
The group identified three types of subsurface changes caused by contact between a glass surface and a foreign object. First, compressive stress during indentation causes structural changes in the glass network, with a slight subsurface densification in the direct physical contact region. Second, tensile stress in the periphery of the indentation causes structural changes, even in regions that are not directly contacted and whose topography has completely recovered. Third, base-catalyzed hydrolysis is more likely in regions where interfacial friction has caused shallow damage.
13
Thus, “invisible” subsurface damage in glass can affect its chemical and mechanical durability. AFM, coupled with IR spectroscopy, can be used to detect and characterize the affected regions. This combination of techniques identifies surface defects and conditions and provides information about the response of a surface to stress. Previous studies focused on macroscopic or microscopic areas of a glass surface, Kim says, but to truly study defects, you have to identify them at their source. “You have to look at the tiny area where the defect started,” he says.
REFERENCES
1.
Kim, S. (Spring 2023), “Helping glass reach its steely potential,” Focus on Materials,
Penn State Materials Research Institute, pp. 46-47. Available
here.
2.
Chen, L., Yang, Y.J., He, H.T., Kim, S.H. and Qian, L.M. (2015), “Effect of coadsorption of water and alcohol vapor on the nanowear of silicon,”
Wear, 332-333, pp. 879-884. Available
here.
3.
Bradley, L.C., Dilworth, Z.R., Barnette, A.L., Hsiao, E., Barthel, A.J., Pantano, C.G. and Kim, S.H. (2013), “Hydronium ions in soda-lime silicate glass surfaces,”
J. Am Ceram. Soc., 96, pp. 458-463. Available
here.
4.
He, H., Qian, L., Pantano, C.G., Kim, S.H. (2014), “Mechanochemical wear of soda lime silica glass in humid environments,”
J. Am. Ceram. Soc., 97, pp. 2061-2068. Available
here.
5.
Surdyka, N.D., Pantano, C.G. and Kim, S.H. (2014), “Environmental effects on initiation and propagation of surface defects on silicate glasses: scratch and fracture toughness study,”
Appl. Phys. A, 116, pp. 519-528. Available
here.
6.
S.H., Liu, H., Kim, S.H. and van Duin, A.C.T. (2020), “Atomistic understanding of surface wear process of sodium silicate glass in dry versus humid environments,”
J. Am. Ceram. Soc., 103, pp. 3060-3069. Available
here.
7.
Lin, Y.-T., Walczak, W., Banerjee, J., Smith, N.J., Agnello, G., Zoba, A.N., Manley, R. and Kim, S.H. (2022), “Particle size and humidity effects on nanocellulose adhesion to glass: Implications for mitigating particulate contamination in industrial processes,”
ACS Appl. Nano Mater., 5, pp. 17004-17011. Available
here.
8.
Luo, J., Huynh, H., Pantano, C.G. and Kim, S.H. (2016), “Hydrothermal reactions of soda lime silica glass–Revealing subsurface damage and alteration of mechanical properties and chemical structure of glass surfaces,”
Journal of Non-Crystalline Solids (JNCS), 452, pp. 93-101. Available
here.
9.
Liu, H., Ngo, D., Ren, M., Du, J. and Kim, S.H. (2019), “Effects of surface initial condition on aqueous corrosion of glass—A study with a model nuclear waste glass,”
J. Am. Ceram. Soc., 102, pp. 1652-1664. Available
here.
10.
Amma, S., Luo, J., Pantano, C.G. and Kim, S.H. (2015), “Specular reflectance (SR) and attenuated total reflectance (ATR) infrared (IR) spectroscopy of transparent flat glass surfaces: A case study for soda lime float glass,”
JNCS, 428, pp. 189-196. Available
here.
11.
Liu, H., Kaya, H., Lin, Y.-T., Ogrinc, A. and Kim, S.H. (2022), “Vibrational spectroscopy analysis of silica and silicate glass networks,”
J. Am. Ceram. Soc., 105, pp. 2355-2384. Available
here.
12.
He, H., Chen, Z., Lin, Y.-T., Hahn, S.H., Yu, J., van Duin, A.C.T., Gokus, T.D., Rotkin, S.V. and Kim, S.H. (2021), “Subsurface structural change of silica upon nanoscale physical contact: Chemical plasticity beyond topographic elasticity,”
Acta Materialia, 208, 116694. Available
here.
13.
Lin, Y.-T., Ogrinc, A.L., Zoba, A.N., Lee, J., Jang, S., Smith, N.J., Banerjee, J., Antony, A., Agnello, G. and Kim, S.H.. (2024), “Revealing ‘invisible’ subsurface structural change/damage in silicate glass made by ‘nearly-elastic contact’ with a spherical smooth surface,”
Acta Materialia, 264, 119571. Available
here.
14.
Lin, Y.-T., He, H., Kaya, H., Liu, H., Ngo, D., Smith, N.J., Banerjee, J., Borhan, A. and Kim, S.H. (2022), “Photothermal atomic force microscopy coupled with infrared spectroscopy (AFM-IR) analysis of high extinction coefficient materials: A case study with silica and silicate glasses,”
Analyt. Chem., 94, pp. 5231-5239. Available
here.
Nancy McGuire is a freelance writer based in Albuquerque, N.M. You can contact her at nmcguire@wordchemist.com.