Wear of alumina-PTFE against non-ferrous substrates
By J.E. Swets, S.M. Harrington and H.S. Khare | TLT Scholarship Research June 2024
Department of Mechanical Engineering, Gonzaga University, Spokane, Wash.
Editor’s Note: This month TLT profiles the 2023 recipient of The E. Richard Booser Scholarship Award, Jackson Swets (Gonzaga University). The scholarship is awarded annually to undergraduate students who have an interest in pursuing a career in tribology. As a requirement for receiving an STLE scholarship, students are given the opportunity to participate in a tribology research project and to submit a report summarizing their research. For more information about the Booser scholarship, visit www.stle.org.
Swets is a third-year student majoring in mechanical engineering and will graduate in May 2025. His research was conducted as a member of the Gonzaga Tribology Lab, and under the mentorship of Dr. Harman Khare. Outside of engineering, Swets likes to play trumpet, ping pong and go mountain biking. You can reach him at jswets@zagmail.gonzaga.edu.
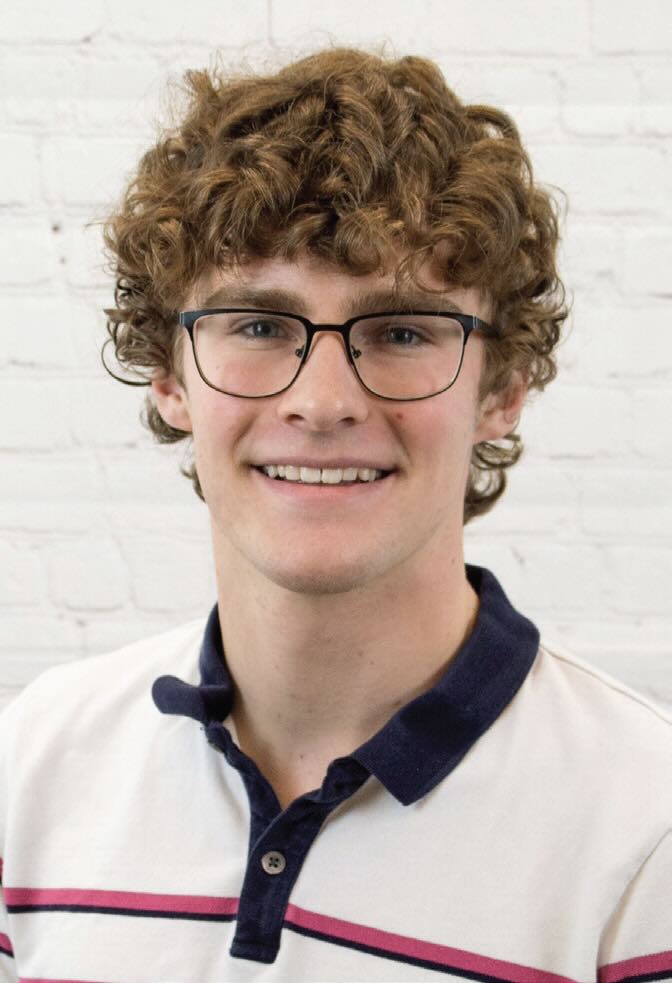
Jackson Swets
Abstract
PTFE matrix composites reinforced with α-Al
2O
3 fillers are a viable solid lubricant which exhibit ultra-low wear rates (k ~ 10-8 mm
3/Nm) when sliding against steel. Low wear in these composites is facilitated by robust tribological films that develop on both sides of the sliding interface. These interfacial films form due to mechanochemical reactions that involve breaking of PTFE chains due to shear stresses developed at the sliding interface, and subsequent passivation by ambient moisture. However, nearly all prior research that reports on ultra-low wear of α-Al
2O
3 PTFE involves sliding against steel substrates. In contrast, some preliminary recent work has shown that alumina-PTFE exhibits high wear against a brass countersurface. These results suggest that ultra-low wear of alumina-PTFE is dependent on counter surface chemistry, however mechanisms for these differences remain unclear. In the present work, the mechanochemical mechanisms that result in differing wear of alumina-PTFE composites on brass and aluminum countersurfaces are examined.
Introduction
Polytetrafluoroethylene (PTFE) has great potential as a solid lubricant due to its low coefficient of friction and chemical inertness, however, unfilled PTFE exhibits high wear under dry sliding
1. The addition of nearly any filler material has been shown to reduce wear rate of unfilled PTFE to varying degrees
1–4. Incorporating alpha-phase alumina nanoparticles (α-Al
2O
3) into PTFE has been shown to reduce wear by nearly five orders of magnitude when sliding against steel
5,6. This dramatic reduction in wear is due to two robust interfacial films that develop under dry sliding of α-Al
2O
3 against steel – one on the composite itself, and the other through transfer onto steel. The film that forms on the steel counter surface is the transfer film, while film that forms on the composite pin itself is the tribofilm. The friction and shear stress of sliding causes the polymer chains in the PTFE matrix to break. The free carbon at the end of the broken PTFE chains reacts with ambient moisture creating perfluoroalkanoic acids. These acids allow for PTFE chains to chelate to the metal oxides both in the steel counter surface, and the alumina within the composite pin
7–9. Although much work has been done in characterizing performance of α-Al
2O
3 PTFE composites, nearly all these studies have utilized stainless steel as the sliding countersurface.
The effects non-ferrous substrates have on the performance of α-Al
2O
3 PTFE composites in dry sliding remain largely uncharacterized. Recent work by Ullah et al. showed that dry sliding against brass results in dramatic increase in wear of α-Al
2O
3 composites, despite the seeming development of a transfer film on the brass counter surface
10. These results beg the following question: does ultra-low wear of the α-Al
2O
3 PTFE system necessarily require the present of steel countersurfaces? In this work, we systematically investigate the effects of non-ferrous countersurfaces on the wear of α-Al
2O
3 PTFE composites to develop a mechanistic understanding of their wear behavior. Furthermore, we propose strategies for controlling wear against non-ferous substrates where alumina-PTFE composite pins are first run on steel to develop a robust tribofilm, and then the same pin is subsequently run on non-ferrous substrates.
2.0 Materials and Methods
2.1 Materials and Sample Preparations
Dry powder PTFE with a reported nominal particle size of 20 μm was purchased from Goodfellow Corp (Pittsburgh, PA). Alpha-alumina nanoparticles with a reported nominal particle size of 27-43 nm were obtained from Nanostructural and Amorphous Materials, Inc. (Katy, TX). Alumina and PTFE powders were combined to yield a mixture with 5 weight percent alumina. Approximately 50 mL of anhydrous ethanol was then added to the mixture, and the mixture was homogenized using a horn sonicator (Branson Sonifier 450). The sonicator operated at 400W and 50% duty cycle for 10 minutes to yield a homogenous solution. The beaker was placed on a hot plate set to 70°C for approximately 12 hours. The beaker remained in ambient conditions under a continuous air stream inside a fume hood for the duration. The dry homogenous powder was transferred to a cylindrical steel mold (inner diameter of 12.7 mm). The mixture was compressed at a sustained pressure of 390 MPa for 20 minutes using a hydraulic press. The cylindrical preform was removed from the mold, wrapped in foil, and placed in a furnace to undergo a programmed sintering. A PID-controlled resistance heater executed a sinter cycle consisting of a ramp to 380°C at a rate of approximately 2°C/min, soak at 380°C for 3 hours, and cool down to ambient temperature at approximately 2°C/min. The sintered cylindrical preform was then milled with a benchtop CNC router (Carbide Shapeoko 3) to nominal dimensions of 6.35 mm × 6.35 mm × 15.5 mm. Multiple pins were independently fabricated using this method and used in testing to facilitate viable replicate wear tests.
Three different materials were used as counter surfaces during wear testing: AISI 304 stainless steel, A6061-T6 aluminum alloy, and 70/30 brass (260 Brass). Test coupons were mechanically polished with 240 grit and subsequently 600 grit SiC bonded abrasive paper. A6061-T6 aluminum alloy coupons were further polished using a rayon cloth with a monocrystalline diamond suspension. Average surface roughness for all countersurfaces were measured to be between 20 and 200 nm before sliding. A scanning white light interferometer (SWLI, Keyence VK-X3000) was used to measure surface roughness.
2.2 Wear Testing
Wear testing was executed on a combination of two custom built linear reciprocating tribometers, with a total of three test stations available for running simultaneous wear tests
(see Figure 1). Composite pins were each mounted to a six-axis load cell (ATI Industrial Automation) using a sample vise. These load cells recorded applied normal force and friction force in real time throughout the sliding cycles. A normal load of 250 N was independently applied at each station using electro pneumaticallyactuated thrusters. This resulted in a nominal contact stress of 6.2 MPa. Prior to testing, composite pins were run-in against 240 grit abrasive paper to ensure conformal contact with the test coupon. Intermittent mass measurements were taken throughout the test for determining wear rate. Mass measurements were taken on a precision analytical balance (Mettler Toledo AT261) with a 10 μg readability.
Wear rate was determined using the modified Archard’s equation:

where
Δm is the measured mass loss, loss, F
n is the applied normal load,
ρ is the density of the composite pin prior to sliding (assumed constant throughout the test), and
D is the cumulative sliding distance.
Figure 1. One of the custom built linear reciprocating tribometers used to execute wear testing. This tribometer allows for two composite pins to be tested simultaneously.
Two different types of tests were executed in this work: ‘pristine’ tests and ‘prefilm’ tests
(see Figure 2). In
pristine (unconditioned) wear tests, composite pins after CNC milling were run against a single steel, brass, or aluminum coupon for 200,000 cycles, interrupted only for intermittent mass measurements. In
conditioning/prefilm wear tests, composite pins were first run against steel for 10, 30, 50, 70, or 200 thousand cycles. Then the same pin (preserving the developed tribofilm, or
prefilm), was run against brass or aluminum for 200,000 additional cycles, also while taking intermittent mass measurements. Wear rates reported for prefilm wear tests refer to values determined from volume loss only during sliding on brass or aluminum (i.e., only after the steel substrate was replaced by a brass or aluminum substrate).
Figure 2. A schematic representation of sequence of steps in (a) pristine/unconditioned wear tests, where composite pins are run against a single substrate for the entirety of the test,and (b) prefilm/conditioning wear tests where an initial period of sliding against steel develops a “prefilm”, which is then run against brass or aluminum.
2.3 Characterization of Worn Interfaces
Morphological, topographical and chemical measurements of the worn interface were carried out using a 3D optical microscope and infrared spectroscopy. Optical and topographic images of the transfer films (on the substrate) and tribofilms (on the composite pin) were collected using a laser confocal microscope (Keyence VK-X3000). This microscope, in the scanning white light interferometer mode, was also used to verify roughness of polished substrates prior to wear testing. Fourier transform infrared (FTIR) spectroscopy, conducted in attenuated total reflectance mode (Thermo Scientific Nicolet™ iS™ 5), of transfer and tribofilms was conducted at the end of wear testing, and at select intervals during the wear test to capture the chemical evolution of the sliding interface.
3. Results and Discussion
Pristine (unconditioned) wear tests of α-Al
2O
3 PTFE against steel, brass, and aluminum were conducted to obtain a baseline measurement against the three substrates. Composite pins in each test were run against polished metal coupons for 200,000 cycles. Interrupted mass measurements were taken throughout the duration. Figure 3 shows representative plots of wear volume as a function of sliding distance for tests run against each of the three metal coupons.
Figure 3. Representative plots of wear volume of α-Al2O3 PTFE against steel, brass and aluminum, as a function of sliding distance. Wear tests shown here were conducted in the unconditioned/pristine configuration.
Wear rate is measured as the slope of linear regression of wear volume plotted as a function of sliding distance (normalized to normal load, following the modified Archard’s equation). There are two distinct regions of wear observed in Fig. 3: initial volume loss and steady state wear. The initial volume loss occurs in the first portion of each test, where the transfer film and tribofilm are developing, before transitioning to a steady state wear regime. This initial volume loss is observed to be much lower on steel, while brass and aluminum have a similar initial volume loss, albeit much higher than on steel. After the films have fully developed, wear rate decreases and remains steady throughout the remainder of the test. Figure 4 shows the steady state wear rate as an average of at least five independent tests for each substrate. The steady state wear of alumina-PTFE on brass and aluminum is seen to be nearly two orders of magnitude greater than steady state wear rate on steel.
Figure 4. Steady state wear rate of alumina-PTFE on steel, brass, and aluminum respectively. Error bars represented variation across replicate wear tests (n>5).
Transfer film development and morphology is known to strongly affect the wear of alumina-PTFE. Topographical images of transfer films for pristine tests on steel, brass and aluminum are shown in Figure 5. The thickness of the steel transfer film is much lower than those on brass and aluminum. This follows conventional wisdom that low wear of polymer composites is correlated with thin transfer films
2. However, brass and aluminum both exhibit similarly high wear (k ~ 10
-5 mm
3/Nm), yet aluminum has a significantly thinner transfer film than that of brass. Brass is also seen to develop a significantly rougher transfer film than aluminum; transfer films on steel are seen to be thinnest and smoothest of the three substrates.
Figure 5. (a-c) Optical micrographs and (d-f) topographic images of the transfer films developed at the end of pristine tests, with α-Al2O3 sliding against steel, brass, and aluminum. (g) height histogram plot for each of the three transfer films shown in (d-f), illustrating the variation in both the mean film thickness, as well as the distribution of heights.
The tribofilms for alumina-PTFE are also characteristically different when run on steel, brass, or aluminum
(Figure 5). Tribofilms chemically consist of carboxylic and hydroxide groups that can be seen as absorbance peaks on FTIR spectra in Fig. 5. When composite pins are run on steel in a pristine test, significant presence of carboxylates and hydroxides is measured. When composite pins are run on brass or aluminum in a pristine test, the presence of carboxylates and hydroxides are significantly attenuated. For composite pins run on brass or aluminum, a tribofilm does initially form but it is worn off during further sliding. Since FTIR spectra are collected at the end of wear test (200,000 cycles), the attenuation of absorbance peaks at wavenumbers corresponding to carboxylate and hydroxide groups are consistent with this observation
(see Figure 6).
Figure 6. (a-c) Representative ATR-FTIR spectra measured on tribofilms run against steel, brass and aluminum substrates, respectively, and (d) spectra obtained for transfer films against the three substrates.
The logical next step is to ask if alumina-PTFE would be able to achieve low wear on brass and aluminum if the tribofilm was present. For prefilm tests, the tribofilm is first developed through conditioning cycles on steel, and then the same pin is subsequently run on brass or aluminum. The number of conditioning cycles was varied. Tests were carried out with 10, 30, 50, 70, and 200 thousand conditioning cycles. Figure 7 plots the number of conditioning cycles on steel vs the subsequent wear rate through 200,000 cycles on brass or aluminum.
Figure 7. Wear rate on brass and aluminum as a function of the number of conditioning cycles on steel.
The dotted line in Fig. 7 represents the wear rate of a pristine test on brass or aluminum for comparison. For brass prefilm tests, on average the developed prefilm typically did not prevent high wear against brass. However, large error bars reflect large variability in measured wear rates, some of whom did in fact exhibit significantly reduced wear rates when compared to pristine wear rate against brass. For aluminum prefilm tests, low wear was consistently achieved for every number of conditioning cycles except 10,000 cycles. This observation is also reflected in the FTIR data. For a prefilm pin after 200,000 cycles on brass, the carboxylate and hydroxide absorbance peaks are much lower than those of the prefilm directly after the conditioning cycles. This signifies that the tribofilm developed on steel was partially worn off, which correlates with high wear. For a prefilm pin (excluding 10,000 conditioning cycles) after 200,000 cycles on aluminum, the carboxylate and hydroxide absorbance peaks are higher than those of the prefilm directly after the conditioning cycles. This signifies that not only was the tribofilm not worn off, but it developed even further. This observation of FTIR absorbance data correlates with low steady state wear.
4. Conclusion
1.
High wear is observed for α-Al2O3 PTFE sliding against brass and aluminum substrates, which correlates with the lack of a robust tribofilm on composite surface, as confirmed by FTIR spectra.
2.
The generation of a prefilm against steel and subsequently running the same pin on brass still results in high wear regardless of the number of conditioning cycles on steel. FTIR spectra support the visual observation of prefilm removal during the initial several sliding cycles against brass.
3.
The generation of a prefilm against steel and subsequently running the same pin on aluminum results in low wear for 30, 50, 70, and 200 thousand conditioning cycles on steel. High wear persists for prefilm tests with 10,000 conditioning cycles, when subsequently run against aluminum.
4.
This study illustrates that brass and aluminum in of themselves might be unable to sustain low wear against α-Al
2O
3 PTFE, however this behavior can be attenuated to varying extents through strategies that involve surface modification of composite pins prior to sliding.
5. Acknowledgement
Jackson E. Swets gratefully acknowledges STLE for the E. Richard Booser Scholarship Award and Gonzaga University for the Steeves-Betzler Student Research Scholar Award. This work was partially supported by the NSF-MRI, award #1919528.
REFERENCES
1.
Biswas, S. K.; Vijayan, K. Friction and Wear of PTFE — a Review.
Wear 1992, 158 (1–2), 193–211.
https://doi.org/10.1016/0043-1648(92)90039-B.
2.
Burris, D. L.; Boesl, B.; Bourne, G. R.; Sawyer, W. G. Polymeric Nanocomposites for Tribological Applications.
Macromol. Mater. Eng. 2007, 292 (4), 387–402.
https://doi.org/10.1002/mame.200600416.
3.
Friedrich, K. Polymer Composites for Tribological Applications.
Adv. Ind. Eng. Polym. Res. 2018, 1 (1), 3–39.
https://doi.org/10.1016/j.aiepr.2018.05.001.
4.
Tanaka, K.; Kawakami, S. Effect of Various Fillers on the Friction and Wear of Polytetrafluoroethylene-Based Composites.
Wear 1982, 79 (2), 221–234.
https://doi.org/10.1016/0043-1648(82)90170-3.
5.
Blanchet, T. A.; Kennedy, F. E. The Development of Transfer Films in Ultra-High Molecular Weight Polyethylene/Stainless Steel Oscillatory Sliding.
Tribol. Trans. 1989, 32 (3), 371–379.
https://doi.org/10.1080/10402008908981902.
6.
Bahadur, S.; Tabor, D. The Wear of Filled Polytetrafluoroethylene.
Wear 1984, 98, 1–13.
https://doi.org/10.1016/0043-1648(84)90213-8.
7.
Krick, B. A.; Pitenis, A. A.; Harris, K. L.; Junk, C. P.; Sawyer, W. G.; Brown, S. C.; Rosenfeld, H. D.; Kasprzak, D. J.; Johnson, R. S.; Chan, C. D.; Blackman, G. S. Ultralow Wear Fluoropolymer Composites: Nanoscale Functionality from Microscale Fillers.
Tribol. Int. 2016, 95, 245–255.
https://doi.org/10.1016/j.triboint.2015.10.002.
8.
Harris, K. L.; Pitenis, A. A.; Sawyer, W. G.; Krick, B. A.; Blackman, G. S.; Kasprzak, D. J.; Junk, C. P. PTFE Tribology and the Role of Mechanochemistry in the Development of Protective Surface Films.
Macromolecules 2015, 48 (11), 3739–3745.
https://doi.org/10.1021/acs.macromol.5b00452.
9.
Pitenis, A. A.; Harris, K. L.; Junk, C. P.; Blackman, G. S.; Sawyer, W. G.; Krick, B. A. Ultralow Wear PTFE and Alumina Composites: It Is All About Tribochemistry.
Tribol. Lett. 2015, 57 (1), 4.
https://doi.org/10.1007/s11249-014-0445-6.
10.
Ullah, S.; Haque, F. M.; Sidebottom, M. A. Maintaining Low Friction Coefficient and Ultralow Wear in Metal-Filled PTFE Composites.
Wear 2022, 498–499, 204338.
https://doi.org/10.1016/j.wear.2022.204338.
Note: This report is excerpted from the manuscript titled “Wear of alumina-PTFE against brass and aluminum: Role of interfacial films and tribochemistry”,
J.E. Swets, S.M. Harrington, and H.S. Khare, Wear (under review).