Meet the Presenter
This article is based on a webinar presented by Michal Michalec and Auburn University on April 8, 2022, titled Large-Scale Hydrostatic Bearing Design and Optimization. The webinar series, titled The Beard Tribology Webinar Series, is named after the late Ralph Beard, who pioneered the study of tribology at Auburn. For more information on the webinar series, visit www.eng.auburn.edu/programs/tribology/beard-tribology-webinar.html.
Michal Michalec is a faculty member in mechanical engineering from the Tribology Research Group of the Institute of Machine and Industrial Design (IMID) at the Brno University of Technology in Brno, Czech Republic, focusing his research on hydrostatic lubrication and machine design. He studies large-scale hydrostatic bearing design. He also studies electro-hydrodynamics with electrosensitive fluids, the so-called electrorheological fluids, and works to modify the friction and film thickness of ionic liquids using electric fields. He also has worked on compressed air powered cars. You can reach Michalec at michal.michalec@vut.cz.
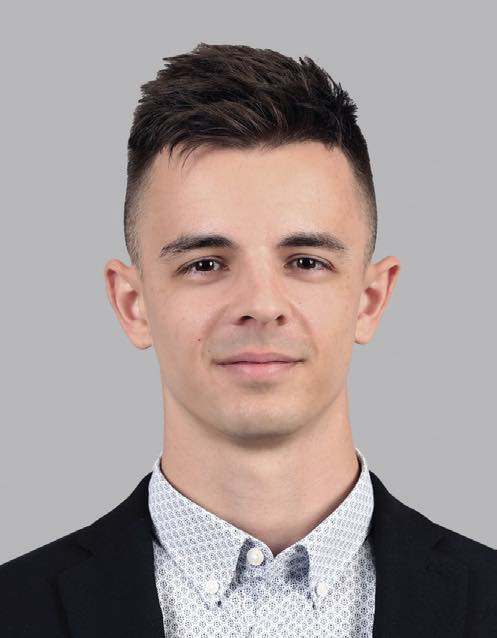
Michal Michalec
KEY CONCEPTS
•
Hydrostatic bearings reduce friction using a pressurized fluid to separate the sliding surfaces.
•
Advantages of hydrostatic bearings include complete separation at zero speed with very low friction and almost no wear, high load capacity and stiffness, very precise motion and the ability to carry large structures.
•
Applications using hydrostatic bearings include telescopes and antennas, heavy machining centers that require precision, heavy turntables (e.g., railways, water gates) and stage or showroom turntables that carry heavy loads.
The function of bearings is to support the relative position of two components, allow their mutual rotary or sliding movement and ensure the transfer of load to the machine frame. Bearings can be rolling, sliding or magnetic
(see Figure 1). Hydrodynamic bearings generate the necessary lubricant film pressure through the relative motion of the moving surfaces and their geometry. Rolling bearings work on nonconformal contacts where higher contact pressures (Hertzian contact) occur. In the case of ball bearings, the friction is further reduced.
Figure 1. Bearings can be rolling, sliding or magnetic. Figure courtesy of Michal Michalec.
In contrast, hydrostatic bearings create the necessary film pressure between the moving surfaces (i.e., the bearing pad and the turntable) through an external source
(see Figure 2). At low speeds, hydrostatic bearings reduce friction even more than hydrodynamic bearings by using a pressurized fluid (e.g., air, oil) to separate the sliding surfaces. The lubricant then flows back from the narrow gap between the surfaces into the hydraulic circuit. Hydrostatic bearings can operate at zero speed and offer very low friction, minimal wear and high stiffness.
Figure 2. Hydrostatic bearings create the necessary film pressure between the moving surfaces through an external source. Figure courtesy of Michal Michalec.
Hybrid bearings combine hydrodynamic and hydrostatic bearings to improve performance, as well as to reduce wear during low-speed operation and start and stop phases.
1
This article is based on a webinar presented by Michal Michalec and Auburn University. See Meet the Presenter for more information.
Hydrostatic bearings
Hydrostatic bearings were initially introduced in 1852 by L.R. Girard and have been further improved by different engineers since then with research continuing today.
Hydrostatic lubrication requires continuous external supply of pressurized lubricant that is generated by the hydraulic circuit
(see Figure 2). The resulting lubricant film thickness is approximately 1-100 micrometers (μm), and the fluid is usually a hydraulic oil (i.e., ISO VG grade 32-68 depending on the environmental conditions), although it can be another fluid (e.g., water, non-Newtonian, air [aerostatic or air bearings]). While the initial cost of hydrostatic bearings is higher than for other bearing types, replacing large rotor rolling bearings with hydrostatic bearings is becoming more common to reduce both system downtime and maintenance costs. In some fields energy consumption has been reduced by as much as 20% through the use of the best available technology in hydrostatic bearings.
1
Calculation of load for hydrostatic bearings
(see Figure 3) is based on Navier-Stok equations. The analytical solution is only for simple geometry systems; however, the calculation can be generalized for more complex configurations and situations.
Figure 3. Calculation of load for hydrostatic bearings. Figure equations courtesy of Bassani, R. and Piccigallo, B. (1992), Hydrostatic Lubrication, Elsevier.
A hydraulic circuit is necessary to supply the pressurized lubricant to the bearing for proper functioning. Single pump bearings are mainly smaller and simpler bearings, while multi-pump bearing systems are much more complex and more frequently used for special applications. The hydraulic circuit is composed of the parts needed to create stable flow of the lubricant to the bearings.
Flow control is used to ensure lubricating film stability and even distribution. With well-aligned bearings the throttle level doesn’t matter; however, with misaligned bearings, the fluid film is not fully carrying the load, and collision of the surfaces leading to damage can occur.
2
Hydrostatic bearings can be divided into types based on load direction: radial, axial or combined, which includes combinations spherical and conical. Pad shape can be 1-recess, multi-recess or multi-pad as illustrated in Figure 4.
Figure 4. Hydrostatic bearings can be divided into types based on load direction. Figure courtesy of Michal Michalec.
Photo courtesy of Michal Michalec.
Hydrostatic bearings offer both advantages and disadvantages depending on the application. Advantages can include:
•
Complete separation at zero speed, in contrast to hydrodynamic bearings
•
Very low friction and almost no wear as there is full separation of the surfaces
•
Damping ability
•
High load capacity and stiffness, depending on the surfaces
•
Very high precision with very precise motion, therefore no stick-slip effect
•
Can be built to carry large structures (e.g., space telescope with 20-meter diameter).
Disadvantages can include:
•
High initial and service costs
•
Continuous pressurized fluid supply required
•
Require more space for the hydraulic circuits than other bearing types
•
Sensitive to manufacturing and assembly errors
•
Increased friction at high speeds, which is why they are combined into hybrid bearings with hydrodynamic bearings at high speeds.
Applications
Applications using hydrostatic bearings include telescopes and antennas
(see Figure 5), heavy machining centers that require precision, heavy turntables (e.g., railways, water gates) and stage or showroom turntables that carry heavy loads.
Figure 5. Applications using hydrostatic bearings include telescopes and antennas. Figure courtesy of Johns, M., Hull, C., Muller, G., et al. (2014), “Design of the Giant Magellan Telescope,” Ground-based and Airborne Telescopes V, Proceedings volume 9145, doi: 10.1117/12.2057286. Available here.
An example of a telescope is the Extremely Large Telescope (ELT) being constructed on top of Cerro Armazones in the Atacama Desert of northern Chile in Antofagasta, Chile (
see Figure 6). The ELT has a 52-meter diameter and is scheduled for completion in 2027.
Figure 6. The Extremely Large Telescope (ELT) is being constructed on top of Cerro Armazones in the Atacama Desert of northern Chile in Antofagasta, Chile. Figure courtesy of ESO and EIE Group.
Research areas
Research is focusing on three main areas:
•
Safety, including collision avoidance, maintenance (off time) minimization and manufacturing and assembly precision
•
Economy, including shape optimization, energy cost reduction and driving force minimization
•
Control, including online diagnostics, maintenance prediction and feedback control.
Figure 7 shows a laboratory experiment studying safety. In the laboratory, the 1.5-meter-long hydrostatic system is fitted with sensors to compare measured data to predictions. Sensors also can be used to align and level the bearings. Research is ongoing on large misalignment to improve performance, support design methodology and compensate for misalignment.
Figure 7. A laboratory experiment studying safety. Figure courtesy of Michal Michalec.
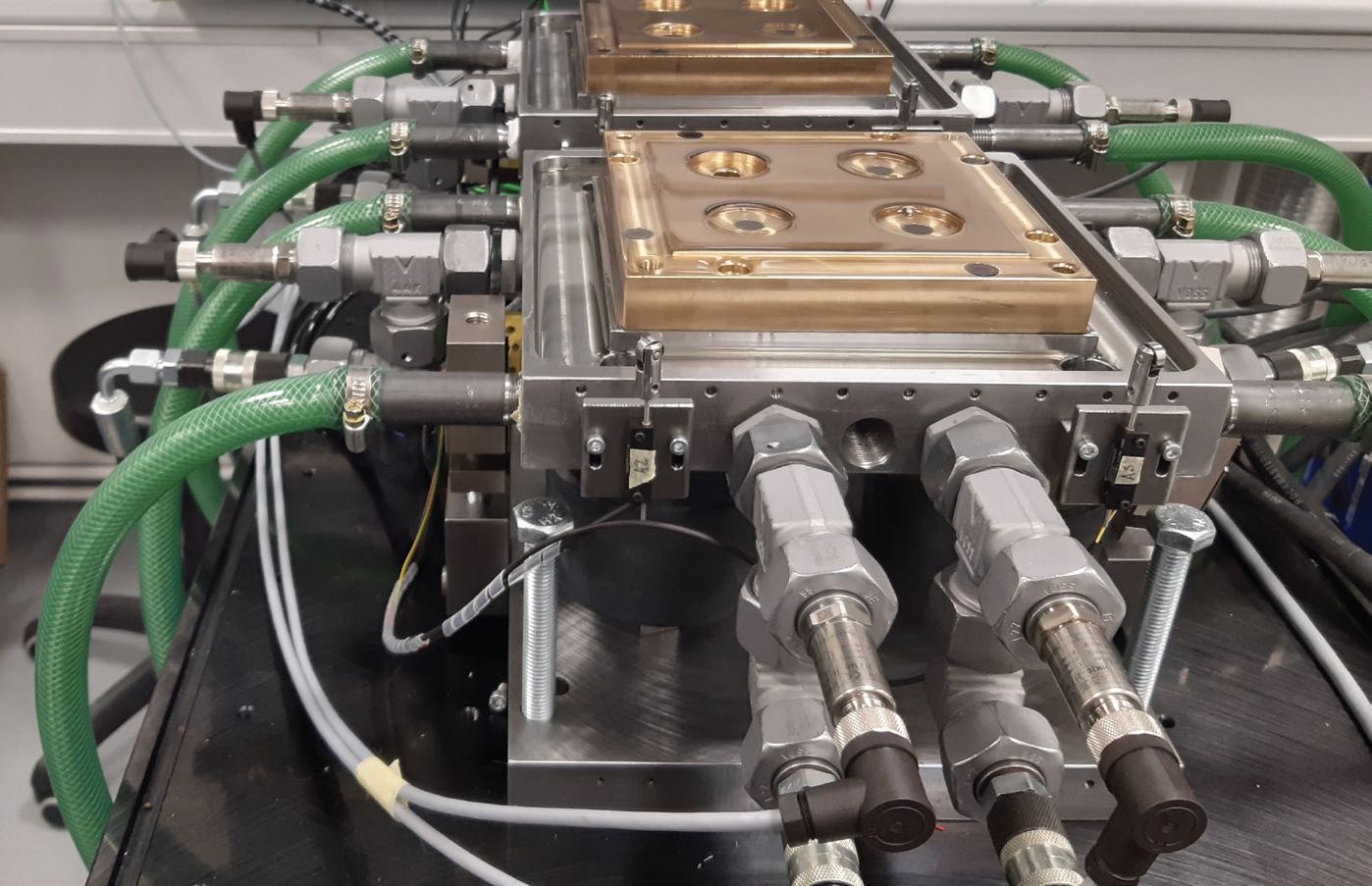
Photo courtesy of Michal Michalec.
Bearing economy research is studying how to reduce pressure loss in certain elements of the bearing system to reduce energy costs. For example, energy consumption by the ELT on an annual basis with 24-hour operation amounts to roughly $115,000 per year. Optimizing the bearing design could result in a 20% reduction in cost (i.e., approximately $23,000 per year). Other economy research involves studying pad shape optimization, which involves design methodology, power loss minimization and computational fluid dynamics (CFD) calibration. Experimental data allow for the calibration of models while studying atypical design shapes.
Bearing control research includes the hydraulic circuit and feedback control systems. Non-symmetric loading and load and small misalignment compensation for high precision and stability applications are other areas of research.
Future research on hydrostatic bearings will look at safety in sliding surface modifications, economy of advanced simulations and adaptive feedback control.
REFERENCES
1.
Michalec, M., Ondra, M., Svoboda, M., et al. (2023), “A novel geometry optimization approach for multi-recess hydrostatic bearing pad operating in static and low-speed conditions using CFD simulation,”
Tribol Lett, 71, https://doi.org/10.1007/s11249-023-01726-3.
2.
Michalec, M., Polnicý, V., Foltýn, J., et al. (2022), “The prediction of large-scale hydrostatic bearing pad misalignment error and its compensation using compliant support,”
Precision Engineering 75, p. 67,
https://doi.org/10.1016/j.precisioneng.2022.01.011.
Andrea R. Aikin is a freelance science writer and editor based in the Denver area. You can contact her at pivoaiki@sprynet.com.