Friction at very small scales
R. David Whitby, Contributing Editor | TLT Worldwide May 2022
Tiny beetles can fly faster than larger insects—but how?
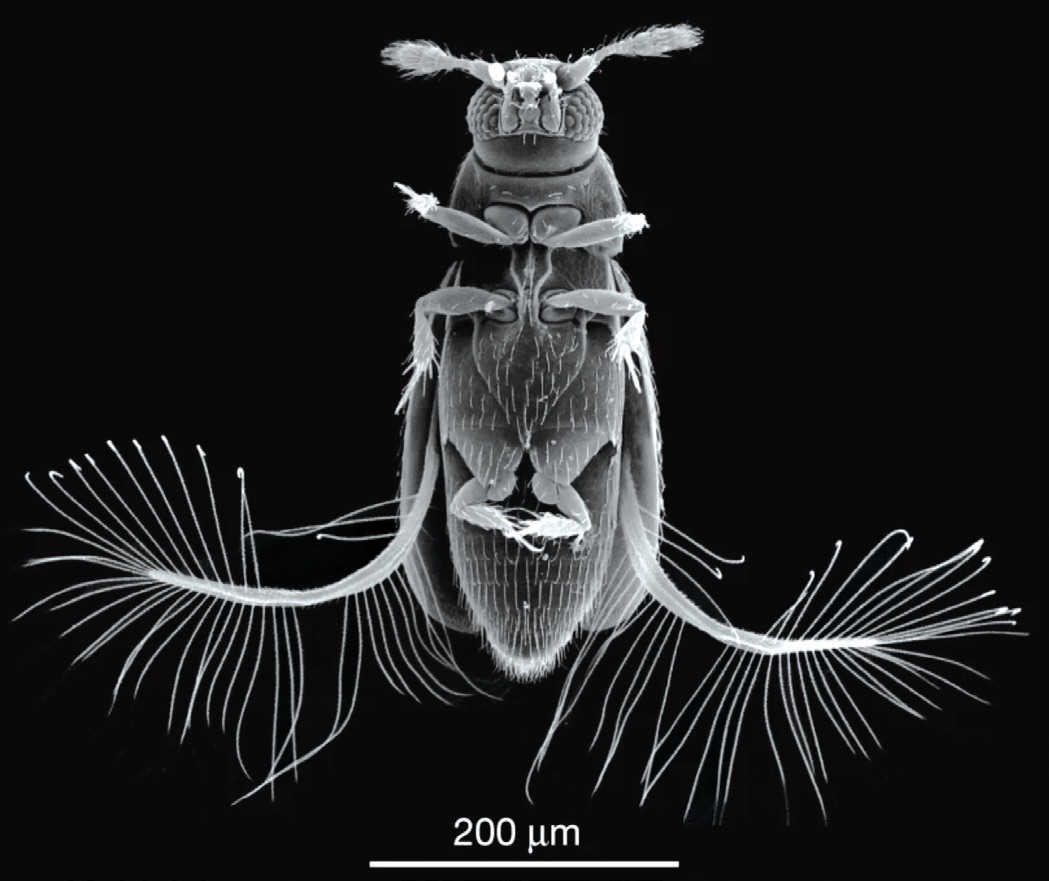
Scanning electron microscopy image showing the relative size of the Paratuposa placentis. Image courtesy of Farisenkov, S.E., Kolomenskiy, D., Petrov, P.N. et al. (2022), “Novel flight style and light wings boost flight performance of tiny beetles,” Nature, 602, pp. 96-100, CC BY 4.0.
The importance of friction to movement depends on where the friction occurs. This was brilliantly illustrated in a paper published in the journal Nature1 in January.
Sergey E. Farisenkov and colleagues wondered how a tiny beetle, Paratuposa placentis (body length of 395 μm) was able to fly at speeds and accelerations of insects three times its size. For an insect that is less than a millimeter long, the frictional resistance of the air becomes a major problem. It is the equivalent of a larger creature trying to fly through water. At such tiny scales, the physics of flight changes, so tiny winged insects have evolved mechanisms to be able to fly.
To find out how the miniature featherwing beetle is able to fly, the researchers constructed a morphological model based on data gained from light, confocal and electron microscopy measurements, a kinematic model using synchronized high-speed videography and a dynamic model using computational methods of solid and fluid mechanics. Combining these techniques provided a comprehensive view of how bristled wings work and explained why common sub-millimeter flying insects have bristled rather than membranous wings.1
The researchers found that the flapping bristled wings follow a distinct figure-of-eight loop consisting of sub-perpendicular up and down strokes, followed by claps at stroke reversals above and below the insect’s body. The modified, hardened forewings of the beetle (known as elytra) act as inertial brakes that prevent excessive body oscillation. Calculations suggest functional decomposition of the wingbeat cycle into two power half strokes, which produce a large upward force, and two down-dragging recovery half strokes. Compared with heavier membranous wings, the motion of bristled wings of the same size requires little inertial power.1 Consequently, muscle power requirements are positive throughout the entire wingbeat cycle, making elastic energy storage unnecessary. These adaptations help to explain how extremely small insects have preserved good aerial performance as they became smaller, one of the factors of their evolutionary success.1
Engineers, physicists, biologists and designers are increasingly interested in investigating the microscopic world, so as to try to miniaturize industrial products to save energy and space. But miniaturization has been occurring in nature for millennia. For more than 300 million years, ecological pressures have been forcing insects to develop extremely small bodies, down to 200 μm long, without losing their ability to fly. As the physical properties of flight depend on size, constraints that are insignificant at the macroscale become significant at the microscale, and vice versa. Compared with larger sizes, flight at small sizes is dominated by viscous air friction rather than inertial forces resulting from the acceleration of the surrounding air. This competition between friction and inertia is key for flight at all size scales and, thus, applies to all animals that move through air.1
Earlier, two-dimensional studies of insect wings’ aerodynamics had revealed that the flow past evenly spaced cylinder lattices reduces aerodynamic force production in bristled wings. Conversely, experiments with mechanical comb-like models suggested slightly larger lift-to-drag ratios during the clap-and-fling phase in bristled wings compared with membranous wings, but these did not cover the full wingbeat cycle. Using the state-of-the-art high-speed videography, it became clear to the researchers that small insects use a wingbeat cycle that is different to that of larger insects.1
Of course, the effects of the frictional resistance of air have been understood by engineers for quite some time. For example, hydrogen gas is used in many medium- and large-sized electricity generators in power stations, because it provides much less frictional resistance to rotors and greater cooling to rotors and stators than air. The ability of Paratuposa placentis to fly as successfully as it does illustrates just how nature has evolved to counter the problem of air-induced friction at tiny scales.
REFERENCE
1. Farisenkov, S.E., Kolomenskiy, D., Petrov, P.N. et al. (2022), “Novel flight style and light wings boost flight performance of tiny beetles,” Nature, 602, pp. 96-100.
David Whitby is chief executive of Pathmaster Marketing Ltd. in Surrey, England. You can reach him at pathmaster.marketing@yahoo.co.uk.