KEY CONCEPTS
•
Cuprous oxide, a potential photocathode that can function as a photoelectrocatalyst to convert carbon dioxide to hydrocarbons, has been found to be unstable when used.
•
To better understand this instability, a cuprous oxide photocathode was evaluated in the aqueous electrolytes, potassium bicarbonate and sodium sulfate, and found to simultaneously oxidize to cupric oxide and be reduced to elemental copper in what is believed to be a photocorrosion process.
•
Introduction of silver nanoparticles with cuprous oxide led to a reduction in photocorrosion, which resulted in a 1.8-fold increase in initial photocurrent density compared to cuprous oxide.
One goal that researchers are working to achieve is to convert carbon dioxide to useful derivatives such as hydrocarbons and even fuels. Mother Nature efficiently reacts carbon dioxide and water through a series of steps to glucose and oxygen in a process known as photosynthesis.
Work is ongoing to develop technologies that emulate photosynthesis. One such project was discussed in a previous TLT article.
1 Giant clams have been found to scatter sunlight to algae, which then converts it to glucose used by the clams in respiration. This synergistic relationship is effective because clams contain optically active cells known as iridocytes that do the light scattering. Researchers took this concept and produced synthetic iridocytes based on silica nanoparticles.
Another technique for converting solar energy to chemical derivatives is through the use of photoelectrochemical (PEC) devices. Dr. Francesca Toma, staff scientist in the Liquid Sunlight Alliance (LiSA) and at Lawrence Berkeley National Laboratory (LBNL) in Berkeley, Calif., says, “PEC devices take light and use it to perform chemical reactions. Light is absorbed efficiently through the use of specific metal oxide photoelectrodes that function as the photoelectrocatalyst. Electrolytes are present in an aqueous solution to facilitate the process.”
The problem with using PEC devices is that they have little to no efficiency and durability. Toma says, “The photocurrent produced when a photoelectrocatalyst converts light to an electric current is usually low. The photoelectrocatalysts involved in the process both at the anode and the cathode are typically not stable and cannot sustain the reaction under typical operating conditions. Either low efficiency is found due to a highly stable material or high efficiency is achieved with an unstable material.”
Toma’s colleague, Dr. Guiji Liu, LiSA and LBNL project scientist, adds: “A tradeoff exists between stability and efficiency. Unfortunately, both are not possible in current PEC devices.”
A potential photocathode to use in the conversion of carbon dioxide to hydrocarbons (also known as the carbon dioxide reduction reaction) is cuprous oxide. Toma says, “Cuprous oxide has been well studied as a photoelectrocatalyst in the production of hydrogen and has suitable properties to harvest electrical energy from visible light. But cuprous oxide is not stable and demonstrates pronounced photocurrent decay within a few minutes.”
Past work suggests that the reason for the decline in performance is due to cuprous oxide simultaneously oxidizing to cupric oxide and reducing to elemental copper. A comprehensive study has now been undertaken to more fully understand the fall off in cuprous oxide’s activity.
Photocorrosion
Toma, Liu and their colleagues monitored the stability of a cuprous oxide photocathode placed in 0.1 molar potassium bicarbonate and 0.1 molar sodium sulfate solutions when illuminated.
Operando ambient-pressure X-ray photoelectron spectroscopy was used at the Advanced Light Source, a national user facility at LBNL, to evaluate how the solid electrode interacted with the aqueous electrolytes.
The researchers detected the presence of cupric and hydroxyl ions in solution. Their formation is dependent upon the electrolyte with faster degradation observed in potassium bicarbonate compared to sodium sulfate.
Toma says, “We found that not only did photogenerated electrons cause the reduction of cuprous oxide to elemental copper, but a positively charged hole acts to oxidize the cuprous oxide to form cupric ions. The latter factor was confirmed because the researchers added an electron scavenger, hydrogen peroxide, to the electrolyte and still observed degradation.”
Toma and Liu believe this is a photocorrosion process. The formation of hydroxyl ions means that the pH of the electrolyte increases. Toma says, “Buffering the electrolyte is a possibility but needs to be done at the local environment near the photocathode. We did not find a way to buffer just the region near the photocathode, but it will be the focus of future studies.”
To mitigate the photocorrosion process, the researchers decided to introduce silver nanoparticles as catalysts used to expedite electron transfer for the carbon dioxide reduction reaction. Liu says, “Silver is a good catalyst for reducing carbon dioxide to carbon monoxide and will complement the ability of cuprous oxide to convert carbon monoxide to hydrocarbons.”
Evaluation of silver and cuprous oxide with acetonitrile, a common solvent used in carbon dioxide reduction, produces approximately a 1.8-fold increase in the initial photocurrent density compared to cuprous oxide. Long-term evaluation showed retention of 57% of the initial photocurrent density when silver is combined with cuprous oxide compared to a 6% retention when only cuprous oxide is present.
The researchers then combined the silver, cuprous oxide photoelectrode with a Z-scheme to facilitate the recombination of holes with electrons. Liu says, “The Z-scheme is an artificial system that is comparable to what occurs in natural photosynthesis where the two photosystems used by a green plant are linked in a ‘Z’-shaped pattern.”
Figure 1 shows a PEC device that the researchers used to react carbon dioxide and water in a photoelectrochemical process to produce ethylene and hydrogen. Faradic efficiencies of approximately 60% for ethylene formation and 40% for hydrogen synthesis were achieved. Toma says, “Faradic efficiency represents the efficiency of the conversion of the (photo)current into actual products during a (photo)electrochemical reaction.”
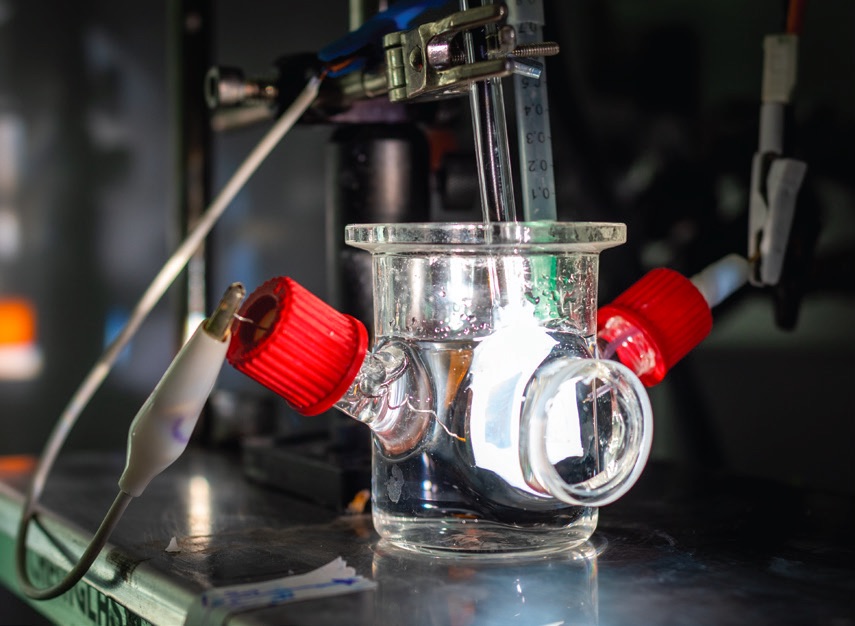
Figure 1. A photoelectrochemical (PEC) device, containing cuprous oxide and silver, converted carbon dioxide and water to ethylene and hydrogen at a Faradic efficiency of 60% for the former and 40% for the latter, respectively. Figure courtesy of Lawrence Berkeley National Laboratory – The Regents of the University of California, Lawrence Berkeley National Laboratory.
Future work will concern gaining a better understanding of the photocorrosion process. Toma says, “We also will be evaluating different electrolytes and trying to determine if higher molecular weight hydrocarbons can be efficiently produced using this technique.”
Additional information can be found in a recent article
2 or by contacting Toma at
fmtoma@lbl.gov.
REFERENCES
1.
Canter, N. (2018), “Maximizing solar energy by emulating natural photobioreactor,” TLT,
74 (3), pp. 16-17. 17. Available
here.
2.
Liu, G., Zheng, F., Li, J., Zeng, G., Ye, Y., Larson, D., Yano, J., Crumlin E., Ager, J., Wang, L. and Toma, F. (2021), “Investigation and mitigation of degradation mechanisms in Cu2O photo-electrodes for CO
2 reduction to ethylene,” Nature
Energy, 6, pp. 1124-1132.