KEY CONCEPTS
•
Heat transfer fluids function by moving heat from one location to another either for the purpose of raising or lowering the temperature in a specific application.
•
Specific types of heat transfer fluids are available to be used in low- and high-temperature applications.
•
The electronics present in an electric vehicle generate high levels of heat requiring the use of heat transfer fluids to ensure they operate at optimum levels for a long period.
•
Direct or immersion cooling is showing potential as a technology that can remove heat from electronics such as battery packs more efficiently than indirect cooling.
The growing use of electronics in many applications is leading researchers to better understand the reasons for using heat transfer fluids. Electronics generate substantial amounts of heat, which needs to be dissipated in order to maintain performance. For the lubricant field, a primary example is the electric vehicle (EV).
EV batteries need to operate at a temperature range between 15 C and 35 C to achieve optimum performance.
1 Heat generation during charge/discharge cycles becomes so intense that it is proving difficult to maintain batteries within that desired temperature range. The result is a loss of battery capacity and potentially a reduction in battery life due to degradation at high temperatures.
Heat transfer fluids are now assuming a more prominent role in EVs due to their ability to quickly remove heat. This article will examine the role of heat transfer fluids in EVs. But before addressing this issue, the use of heat transfer fluids, in general, will be discussed, as they have been employed in industrial applications for a long period of time.
Key industry experts were contacted to obtain their expertise on heat transfer fluids and on thermal management challenges with EVs.
The following experts were contacted.
1. Dr. Bethan Warren, Croda Europe Ltd.
2. Thiago Alonso, Dow Chemical
3. Michael Bates, Duratherm
4. Dr. Serya Dutta, Dynalene
5. Dr. Satish Mohapatra, Dynalene
6. Dr. Babak Lotfi, ExxonMobil Chemical
7. Alex Sammut, The Lubrizol Corp.
8. Edward Cass, Paratherm
9. Ryan Ritz, Paratherm
Functions of a heat transfer fluid
Michael Bates, technical director for Duratherm in Lewiston, N.Y., says, “The basic function of a heat transfer fluid is to move heat from one location to another. This process either leads to heating or cooling a specific system in a particular application.”
Edward Cass, technology manager for Paratherm, division of The Lubrizol Corp. in King of Prussia, Pa., indicated that heat transfer fluids are used to indirectly, uniformly and efficiently transfer or move energy in the form of heat from one region to another at low system pressures. He says, “Most often, the fluid is circulated through a heating or cooling source and, subsequently, through a process loop that contains one or more thermal process units. These units may include tanks, reactors, kettles, rollers, dryers, ovens, dies, etc.”
Thiago Alonso, technical services and development scientist and global innovation leader for energy and heat management at Dow Chemical in Lake Jackson, Texas, says, “The main function of a heat transfer fluid is to remove heat from a sourcing point and supply it to different consumers such as reactors, extruders, distillation and extraction towers, among many other applications that demand heat for proper operation. Heat transfer fluids operate in an efficient way with reduced risks of handling fuels in a centralized and controlled unit, run at lower pressures compared to steam, exhibit low flammability and excellent process and temperature control.”
Dr. Satish Mohapatra, president & CEO of Dynalene in Whitehall, Pa., says, “The main function of a heat transfer fluid is to transport thermal energy from one component of a system to another. It also transfers the heat into or out of the component, thereby either heating or cooling it.”
High-temperature versus low-temperature functions
Ryan Ritz, global director of technology for Paratherm, states that the functions of heat transfer fluid operating at high temperature versus low temperature are no different. He says, “The difference between fluids suitable for one or the other is contingent on physiochemical properties advantageously exploited for their intended use. For example, low-temperature fluids will usually have low viscosities, higher vapor pressures and narrower boiling ranges as compared to high-temperature fluids.”
Mohapatra contrasts high-temperature heat transfer fluids with low-temperature fluids. He says, “High-temperature heat transfer fluids are primarily used for heating a system component, whereas low-temperature fluids are primarily used for cooling the components. In certain cases, a high-temperature fluid can be used to cool a system component by removing the heat from it. Similarly, a low-temperature fluid can be used to heat a component that is very cold.”
Alonso feels that the distinction between high and low temperature is somewhat artificial. He says, “The terms are used in general to distinguish between mineral oil, organic and silicone-based fluids and glycol/water-based fluids. In general, glycol/water-based fluids exhibit advantages over other chemistries in the 25 C to 150 C operating range due to their lower cost and superior physical properties. Certain silicone and organic fluids can be used at operating temperatures less than -25 C and down to -100 C where it is not practical to use glycol/water-based fluids due to their higher viscosities and the possibility of use temperatures being below the glycol-water freezing points.”
Alonso continues with a discussion of high-temperature heat transfer fluids. He says, “Mineral oils, synthetic oils and silicones are typically used at temperatures above 150 C and up to 400 C. They can be used in the glycol-water use temperature range but typically are not cost effective. However, there may be situations, for example, where a process will require the use of an organic or silicone-based fluid in the -25 C to 150 C temperature range due to water reactivity with the process material. The choice of fluid chemistry in this temperature range will depend primarily on the maximum desired use temperature and on fluid physical properties, environmental profile and cost.”
Bates indicates that requirements for low-temperature heat transfer fluids are different than for high-temperature heat transfer fluids. He says, “Low-temperature heat transfer fluids can range from as low as -80 C to approximately 150 C. One of the most important properties for low-temperature heat transfer fluids is viscosity. They must display low enough viscosity, ideally to achieve turbulent flow, to be useful in cooling or keeping systems such as chillers operating at their desired temperature.”
In contrast, high-temperature heat transfer fluids must avoid degradation by mechanisms such as oxidation and thermal breakdown, according to Bates. He adds, “Factors such as the heat source and whether the flow of the heat transfer fluid is turbulent have to be considered in evaluating a high-temperature heat transfer fluid.” Degradation issues due to overheating (coked heaters) and oxidation (sludged expansion tank) are shown in Figures 1 and 2.
Figure 1. Overheating is one cause of heat transfer fluid degradation as shown by this image of a coked heater. Figure courtesy of Duratherm.
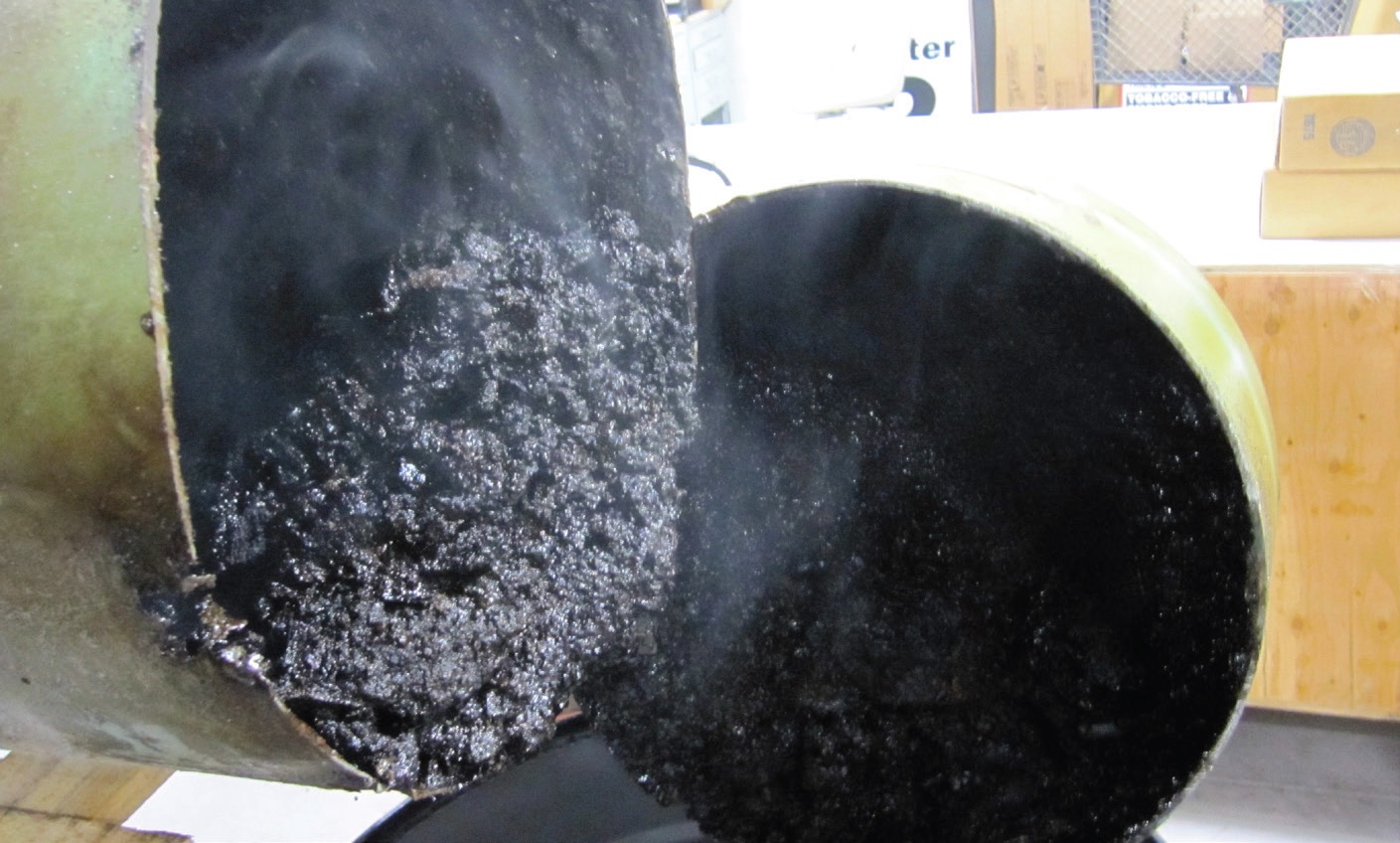
Figure 2. Heat transfer fluids can oxidize during use, which will lead to premature failure as shown by this image of a sludged expansion tank. Figure courtesy of Duratherm.
Examples of heat transfer fluids
Mohapatra divides the types of heat transfer fluids into low temperature and high temperature types. He says, “High-temperature heat transfer fluids range from aromatic and aliphatic hydrocarbons to silicones and, in some cases, molten salts. Low-temperature fluids can be aromatic and aliphatic hydrocarbons, glycols, alcohols, salt brines and silicones.”
Bates distinguishes the high temperature from the low temperature types of heat transfer fluids. He says, “Many of the high-temperature heat transfer fluids are hydrocarbon or synthetic based that can operate at elevated temperatures, though not all of them are oxidatively stable. Aromatic-based fluids such as hydrogenated terphenyls are more likely to be stable at very high temperatures. Water glycols are the most commonly used low-temperature heat transfer fluids but are not able to achieve efficient cooling below -18 C because they tend to get thicker and can get slushy. For lower temperatures, silicones, alcohols, special hydrocarbon fluids and some aromatic-based fluids need to be used.”
Cass classifies heat transfer fluids into aqueous, mineral oil and synthetic categories. He says, “The most common heat transfer fluid in the world is water because its unique properties make it efficient for heating and cooling but limit its practical operating range. Water glycol blends and brine solutions extend the operating capabilities of aqueous fluids but still have limitations.”
Cass continues, “High-temperature fluids may include both natural organic hydrocarbon and synthetic chemistries. Natural organic fluids are generally suitable for operation from 120 C to 300 C. Fluids formulated with Group II and Group III base oils are usually superior in terms of thermal stability and fouling potential versus their Group I counterparts. For operating temperatures in excess of 300 C, synthetic aromatics are preferred. There are many options available in this fluid class, including alkylated benzenes, alkylated biphenyls, aryl ethers, hydrogenated terphenyls and diphenyl ethane blends.”
Cass states that low-temperature fluids are able to achieve minimum operating temperatures as low as -115 C. He says, “Low-temperature fluid types include glycols, silicones, synthetic hydrocarbons, ethers, esters and fluorinated hydrocarbons.”
Selection criteria
Bates outlines at least several parameters that need to be considered in selecting a heat transfer fluid. He says, “For high-temperature properties, the flash point of the fluid is often desired to be greater than the operating temperature. The most important consideration is the bulk fluid temperature in a particular application as well as the skin or film temperature. Other parameters to evaluate include the physical properties such as heat capacity, density and viscosity. The heat transfer coefficient, which takes all of the properties of a fluid into consideration, also is an important parameter that should be determined.”
Bates continues, “Safety criteria, such as toxicity, are important for low-temperature heat transfer fluids. Above all, a user selecting a heat transfer fluid for a specific application must not look at just one property.”
Ritz indicates that no “one-size-fits-all” solutions exist for heat transfer fluids. He says, “The fluid should be matched to meet or exceed the minimum and maximum operating temperatures and minimum startup temperatures of the process. A properly specified fluid also is matched to maximize heat transfer efficiency and safety of the operation. Therefore, physical properties such as viscosity, density, specific heat, thermal conductivity, boiling range and vapor pressure should all be considered and optimized for an efficient process.”
Mohapatra lists seven criteria that need to be followed in picking a heat transfer fluid. He says, “Criteria for selecting a heat transfer fluid include temperature range, thermophysical properties, toxicity, flammability, environmental qualities, availability and cost.”
Alonso’s input on selection criteria include maximum temperature of use, pressure, degradation profile, health and safety concerns, flammability, odor and, of course, cost.
Maintenance practices
Alonso discusses maintenance practices that apply to high-temperature and low-temperature heat transfer fluids. He says, “The manufacturer’s maximum use temperature guidelines should not be exceeded. Use above the maximum recommended operating temperature can result in excessive fluid degradation and, potentially, damages to processing equipment due to fouling/coking. For fluids operating at elevated temperatures, exclusion of oxygen is critical to ensure long fluid life. Fluid condition should be periodically monitored to keep fluid within recommended operating parameters. Levels of degradation products such as low and high boiling compounds also need to be monitored. For glycol/water-based fluids, the focus should be on analysis of the condition of the glycol concentration and inhibitor package.”
Alonso states that many fluid suppliers offer a fluid analysis program to monitor the condition of the fluid and make recommendations for fluid maintenance.
Mohapatra believes that sample testing by the fluid manufacturer needs to be done periodically to ensure the health of the heat transfer fluid. He says, “Air/oxygen, high temperature and moisture are the biggest threats to most of the heat transfer fluids. Care should be taken to have an inert head space and prevent thermal runaways.”
Water-glycol heat transfer fluids also can be vulnerable to foaming during use. Dr. Serya Dutta, R&D manager for Dynalene, says, “Foaming in water-glycol heat transfer fluids can be caused by the presence of contaminants, certain supplemental coolant additives and the presence of air in the system due to cracks or leaks. Addition of defoamers can suppress the foaming tendency of the heat transfer fluid, which could have otherwise led to problems during filling a system or operation. It is recommended to inspect the health of an aging heat transfer fluid to make sure that the defoamers are not depleted, no contaminants or debris are present and the heat transfer system is not facing any mechanical issues.”
Dutta recommends that ASTM D1881 be used to evaluate the foaming potential of a water-glycol heat transfer fluid. Figure 3 shows how a specific heat transfer fluid can generate foam during a test.
Figure 3. The experimental setup for evaluating the foaming of a water-glycol heat transfer fluid using ASTM D1881 is shown. Figure courtesy of Dynalene.
Cass feels that proactive system maintenance is key to maximizing fluid performance over time. He says, “Fluid lifetime is a function of many real-world variables, which makes it critical that all system components—fluid, heaters, chillers, pumps, valves, etc.—be properly maintained
according to OEM recommendations. Deviation of physical properties outside of established optimal ranges should be investigated and corrected to ensure fluid longevity and safety. Heat transfer systems should be routinely assessed to ensure temperatures, pressures and cycle times are continuously stable. Thermal fluid leaks should be addressed and corrected with haste as they can lead to dangerous situations.”
Maintenance of heat transfer fluids is very important because there are typically no visible signs of degradation until actual failure, according to Bates. He says, “Heat transfer fluids have operating timelines that can be as small as 3,000-5,000 hours and as long as 12-15 years depending upon the application. During this time, analysis of such parameters as acid number, viscosity and flash point are important to assess the condition of the heat transfer fluid. Operators need to keep a history of analyses done on a specific system to monitor the condition of the heat transfer fluid and determine if corrective actions are needed to prevent failure.”
Important applications
Ritz included not just applications but also specific process equipment that may use heat transfer fluids. He says, “Heat transfer fluids are used in a plethora of applications including oil and gas refining, chemical processing, polymer manufacturing, industrial drying processes, food and beverage processing, pharmaceutical processing, biomass processing, extraction sciences, electronics cooling, steam generation, etc. Specific process equipment utilizing heat transfer fluids may include tanks, jacketed vessels, reactor vessels, reboilers, distillation towers, thermal oxidizers, autoclaves, calendar rolls, platen presses, molds and dies, dryers, ovens and fryers.”
Bates highlighted the use of heat transfer fluids in food and pharmaceutical applications and, in general, chemical manufacturing.
Alonso points out that any unit operation in the chemical engineering world will most likely use heat transfer fluids as the most efficient way to transfer and consume heat in different processes. He includes the following list of applications: liquid natural gas production, refineries, synthetic fiber production, polyethylene terephthalate resin production, petrochemicals, HVAC, climate chamber, food and beverage processing and many others.
Mohapatra contributed the following applications: beverage production, ice rinks, cold storage, electronics and automotive.
Renewable energy sources
Heat transfer fluids are assuming a large role in the generation of energy from renewable sources. The contributors were asked to provide input on solar energy, geothermal energy and thermal energy storage.
Alonso says, “Heat transfer fluids are used in several solar applications. Water-glycol fluids have been used in roof top-mounted solar collectors to provide heat for domestic hot water heaters and hydronic heating systems. Synthetic aromatic-based fluids have been used for a number of years in parabolic trough concentrating solar power plants. In this application, the heat transfer fluid is heated to approximately 400 C in the parabolic solar collectors and used to produce superheated steam that can be used to drive a turbine generator that produces electricity.”
Mohapatra says, “Thermal energy storage is very important in the concentrated solar power (CSP) plants in order to produce electricity when the sun is not shining. Heat transfer fluids also are used to transport the thermal energy obtained from the solar collectors to the component where it is used to generate electricity and also to charge the thermal energy storage system. Hydrocarbon mixtures with very high temperature stability (biphenyl/diphenyl oxide mixture) are typically used as heat transfer fluids up to 390 C, and nitrate-based molten salt mixtures are used as a thermal storage medium.”
Cass says, “In CSP-generation applications, a configuration of mirrors concentrates the sun’s energy onto some type of receiver where heat transfer fluids are circulated to transport that thermal energy into a power generator (turbine). The two most common CSP technologies utilizing heat transfer fluids are parabolic troughs and ‘power towers.’ Due to the extremely high energy output from the sun, CSP applications demand the use of a high-temperature, synthetic-based heat transfer fluid, usually a vapor phase fluid designed to maximize temperature capacity.”
Cass indicates that thermal energy storage uses a molten-salt-based fluid medium. He says, “The reason is that salts display very low rates of heat loss over time and still can be circulated with the proper system technologies. The importance of thermal energy storage in CSP systems is to ensure that energy production is maintained when there is no daylight.”
There is a greater use of water-based heat transfer fluids in geothermal energy applications because energy transfer occurs at very moderate and consistent temperatures. Cass says, “Demand for thermal stability of the heat transfer fluid is not as high as CSP. Glycols, brines and even alcohol-based fluid formulations are common in geothermal heat pumps.”
Bates confirmed that extreme high-temperature, aromatic-based heat transfer fluids operating in the vapor phase are critical in extracting solar energy from concentrated collectors. He says, “Another side to this is thermal energy storage, which acts as a thermal battery to conserve energy until it is needed.”
Water-glycol heat transfer fluids are mainly used in a loop in geothermal applications. Bates says, “Unfortunately, this energy source has not taken hold in the marketplace with the emphasis for heat transfer fluids placed on solar power.”
Effectiveness of heat transfer fluids
Ritz feels that the current heat transfer fluids are effective as long as they are monitored while in use to ensure optimum long-term performance. He says, “Heat transfer fluids all degrade over time when exposed to thermal energy. The ability to monitor the relative rates of degradation and take action quickly are key to not only extending the life of the fluid but, more importantly, preventing the accumulation of degradation products that can interfere with proper system functionality. Any heat energy that is not transferred from the heat source to the process unit can ultimately be used to break down the fluid molecules; therefore, more efficient fluid (based on thermophysical property ratios) will inherently last longer.”
Alonso agrees, “Fluids can be effectively used for extended periods of time (years) when properly maintained and operated within the fluid manufacturer’s recommended operating conditions. Exceeding maximum use temperature should especially be avoided. Thermal stability, or fluid life at a given set of conditions, is determined by the molecular structure of the components comprising the heat transfer fluid. The maximum use temperature of a particular fluid is a measure of the fluid’s thermal stability. A fluid with a maximum recommended use temperature of 400 C that is used in a system operating at 300 C will undergo very little degradation and have a very long service life. That same fluid operating at 410 C will have a far shorter life, exponentially shorter in fact.”
Alonso points out that aromatic hydrocarbon and polydimethylsiloxane-based heat transfer fluids are limited in operational temperature to between 400 C and 425 C. For higher use temperatures, he indicates that eutectic mixtures of inorganic salts are practically the only alternative.
Future trends
One common theme among the respondents is that the future heat transfer fluids will be developed with health and safety and sustainability in mind.
Bates says, “There is a need to identify more environmentally friendly, high-temperature heat transfer fluids that are less toxic than the current technology based on aromatic chemistry.”
Alonso also agrees that more ecofriendly fluids with better environmental, health and safety profiles are needed. He adds, “Heat transfer fluids with higher maximum use temperatures are desired mainly for CSP units.”
Cass says, “The traditional goal for development of superior heat transfer fluids has always been the optimization of cost, performance and service life. Current and future demands are calling for the heat transfer fluid industry to look deeper into sustainability of materials and the ability to customize performance capabilities depending on the specific needs of niche applications. This will lead to some more demanding innovations than the ‘one-size-fits-most’ style of industrial heat transfer fluids that dominates the market today. As technologies advance in the world around us, the demand for more specialization and customization of heat transfer fluids will increase as well. This will likely lead to fluid providers working more collaboratively with OEMs and end-users to design bespoke solutions.”
Function of a heat transfer fluid in an electric vehicle
Excessive heat generated by an EV during use has been found to reduce the operating life of key components such as the electric motor and the battery. STLE member Dr. Babak Lotfi, product development technologist at ExxonMobil Chemical Co. in Baytown, Texas, indicates that EV design has rapidly evolved to the point where the electric motor poses challenges from both thermal management and tribological standpoints. He says, “Many automotive manufacturers have been adopting the integrated drive-unit design. In this approach, a single fluid is used for cooling the electric motor and electrical components in addition to lubricating gears, bearings and possibly clutches in the drive unit. This design provides more efficiency due to the EV’s smaller size and reduced weight. In this design, the drive-unit fluid is typically a hydrocarbon (such as a synthetic polyalphaolefin, PAO) which is being used for direct cooling and lubrication, and it can potentially provide superior thermal management compared to water-glycol indirect cooling methods.”
Dr. Lotfi feels that the battery may require advanced thermal management to optimize safety, performance and potentially driving range. He says, “Different options that can be used for battery thermal management include air, water-glycol fluids, dialectic (e.g., hydrocarbon) fluids, phase-change materials and refrigerants. Dielectric fluids can provide a direct cooling approach that offers a more uniform thermal management distribution in the system potentially leading to improved safety, thermal management, performance and extending the life of the battery in the EV.”
Dr. Bethan Warren, lead application scientist, energy technologies, Croda Europe Ltd. in Snaith, UK, says that thermal management is a key consideration for an EV in the same way that it is in an internal combustion engine. She says, “Components in both engines generate heat and have limited endurance at high temperatures. Therefore, heat transfer fluids are utilized in order to remove heat as well as manage component temperatures to keep them at their optimum for best efficiency and longevity.”
Warren continues, “An EV is generally made up of a battery, an electric motor, some form of transmission and power electronics. Each of these components may generate heat and the ambient temperature, as well as the function the EV is performing (e.g., driving or charging), can have rapid and significant influences on component temperature. Heat transfer fluids are used to manage these temperatures and enable each of these components to operate at their optimum temperature.”
As an example, Warren states that a lithium-ion battery operates optimally at a narrow temperature range discussed earlier in the article. At higher temperatures, performance degradation can occur and the risk of thermal runaway increases significantly. Charging also can be impaired as the electrical resistance increases as the temperature increases. At lower temperatures, poor performance, reduced range and reduced charging ability will occur. Circulation of the heat transfer fluid through the battery compartment will minimize the chance of the battery being exposed to either high or low temperatures.
Alex Sammut, senior manager, global electronic vehicle applications for the Lubrizol Corp. in Wickliffe, Ohio, believes heat transfer fluids are needed to protect electronics during operation and to facilitate the development of high-speed charging. He says, “During normal operation, EV systems will generate heat, which needs to be managed to prevent damage to batteries and electronics. As charging times decrease, the need for temperature control becomes essential. High-speed charging in excess of 300 kilowatts of power is spreading globally. Even with high efficiency charging, a significant amount of heat (>12-15 kilowatts) is generated and must be managed.”
Direct or immersion cooling
The potential for an EV to generate high levels of heat means that an alternative to the indirect cooling used in an internal combustion engine is required. A logical approach is to place the heat transfer fluid directly in contact with the sensitive components in an EV in a process known as direct or immersion cooling.
Alonso says, “Current battery packs are cooled by having the heat travel from the battery through a heat conductive compound to a heat exchanger that rejects the heat to the heat transfer fluid. The heat transfer fluid is circulated to a radiator where the heat is rejected to air. Direct or immersion cooling would have the heat transfer in direct contact with batteries, eliminating the heat conductive compound and the heat exchanger.”
Warren defines indirect and direct cooling. She says, “Indirect cooling utilizes a coolant circuit with cooling plates or jackets. These cooling components are filled with the cooling fluid, and the plates are thermally bonded to the components to be cooled. Heat generated by the component must flow through the bonding, through the plate and into the cooling fluid before being taken away.”
Warren reveals that direct cooling is more efficient than indirect cooling because the path the heat has to take is much shorter, and heat can be removed at the source. Due to this greater efficiency, the temperature across a component is more homogeneous when using direct cooling. Indirect cooling needs additional components, including the cooling plates that require more metal, more mass and volume, which is undesirable to optimize the efficiency of an EV.”
To evaluate the differences between direct and indirect cooling, a model battery module based on cylindrical cells was created and used for testing
(see Figure 4a and 4b). The direct or immersion cooling set up is shown in Figure 4a, while a cold plate cooled scenario is shown in Figure 4b.
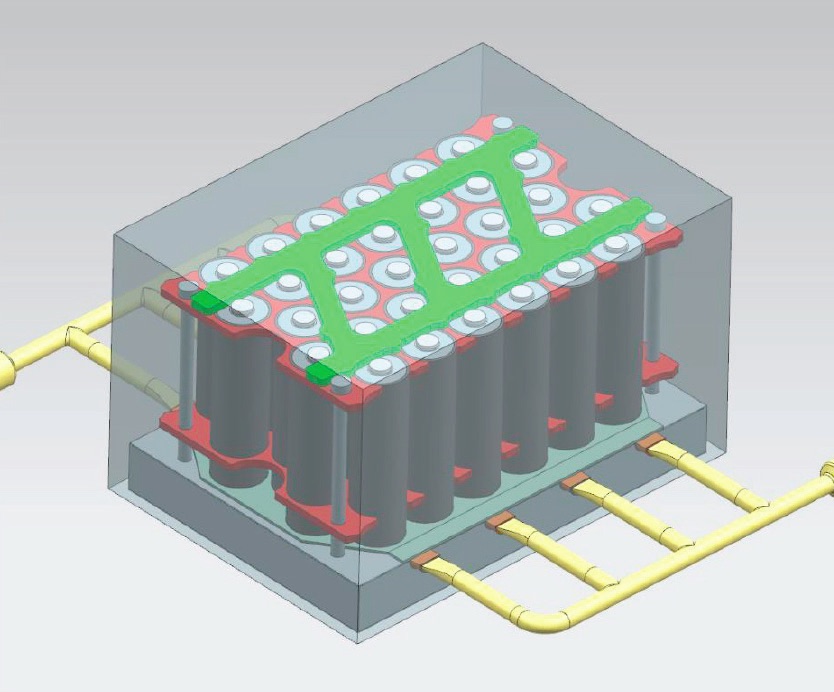
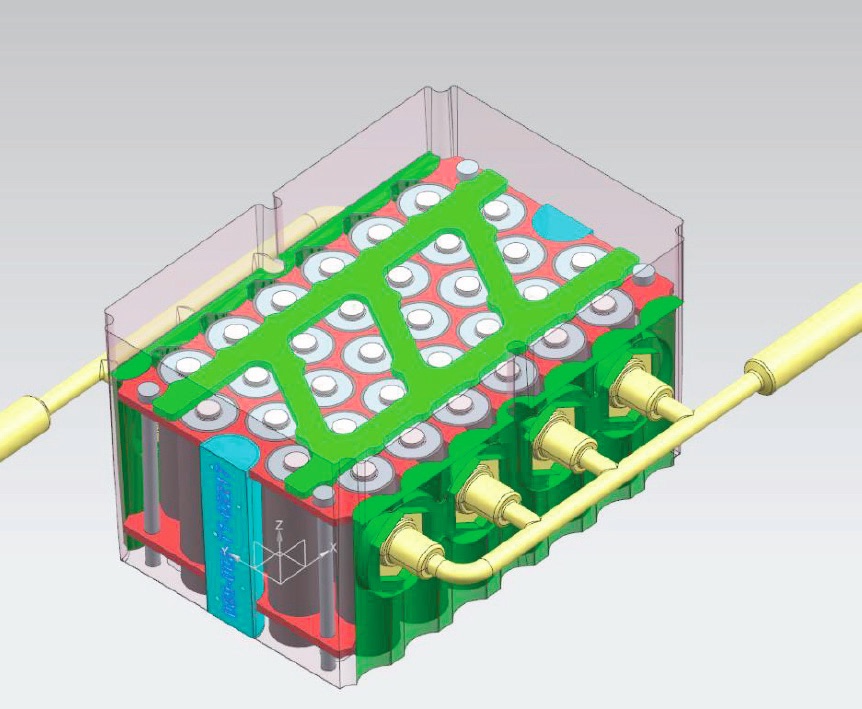
Figure 4. A model battery module based on cylindrical cells is shown for direct or immersion cooling (a) and for indirect cold plate cooling (b). Figure courtesy of D2H Engineering.
Warren says, “Across the flow rates tested, the maximum temperature of the immersion cooled cells were on average 20% lower than the indirect system, and, in addition, the temperature variation across a given cell was reduced by approximately 50% when immersion cooling was used, meaning the cell had better homogeneity.”
Figure 5 shows the maximum battery cell temperature for direct versus indirect cooling at three different flow rates. As the flow rate increases, the difference between direct and indirect cooling also increases. Temperature variations within the battery cell for direct versus indirect cooling are shown in Figure 6. The benefit of direct cooling is shown by the lower temperature variation at the three flow rates.
Figure 5. The maximum battery cell temperature for immersion cooled (direct) versus cold plate cooled (indirect) at three cooling rates is shown. Figure courtesy of Croda/D2H Engineering.
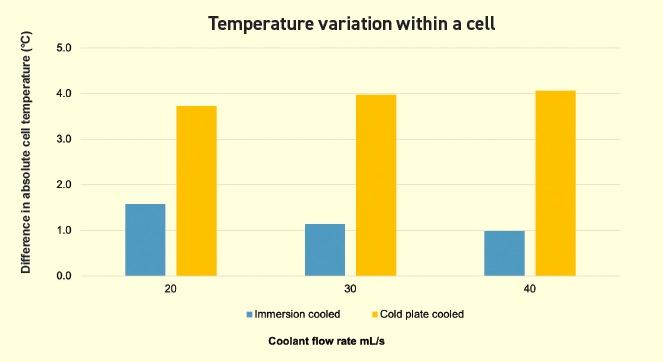
Figure 6. Temperature variations within the battery cell for immersion cooled (direct) versus cold plate cooled (indirect) at three cooling rates indicates that direct cooling leads to a more uniform temperature, which is favored to extend battery life. Figure courtesy of Croda/D2H Engineering.
Sammut indicates that with direct cooling, the heat transfer fluid is in direct contact with the battery and electronics allowing for a greater cooling surface area with the hottest parts of the EV system. A schematic showing direct cooling is shown in Figure 7.
Figure 7. This schematic shows the benefits of direct cooling where the heat transfer fluid is in direct contact with the battery and electronics. Figure courtesy of The Lubrizol Corp.
In contrast, indirect cooling systems separate the fluid, typically water-glycol, from electronic components. Figures 8 and 9 show two examples of indirect cooling: side and bottom tray cooling.
Figure 8. A schematic shows the indirect cooling method known as side cooling. Figure courtesy of The Lubrizol Corp.
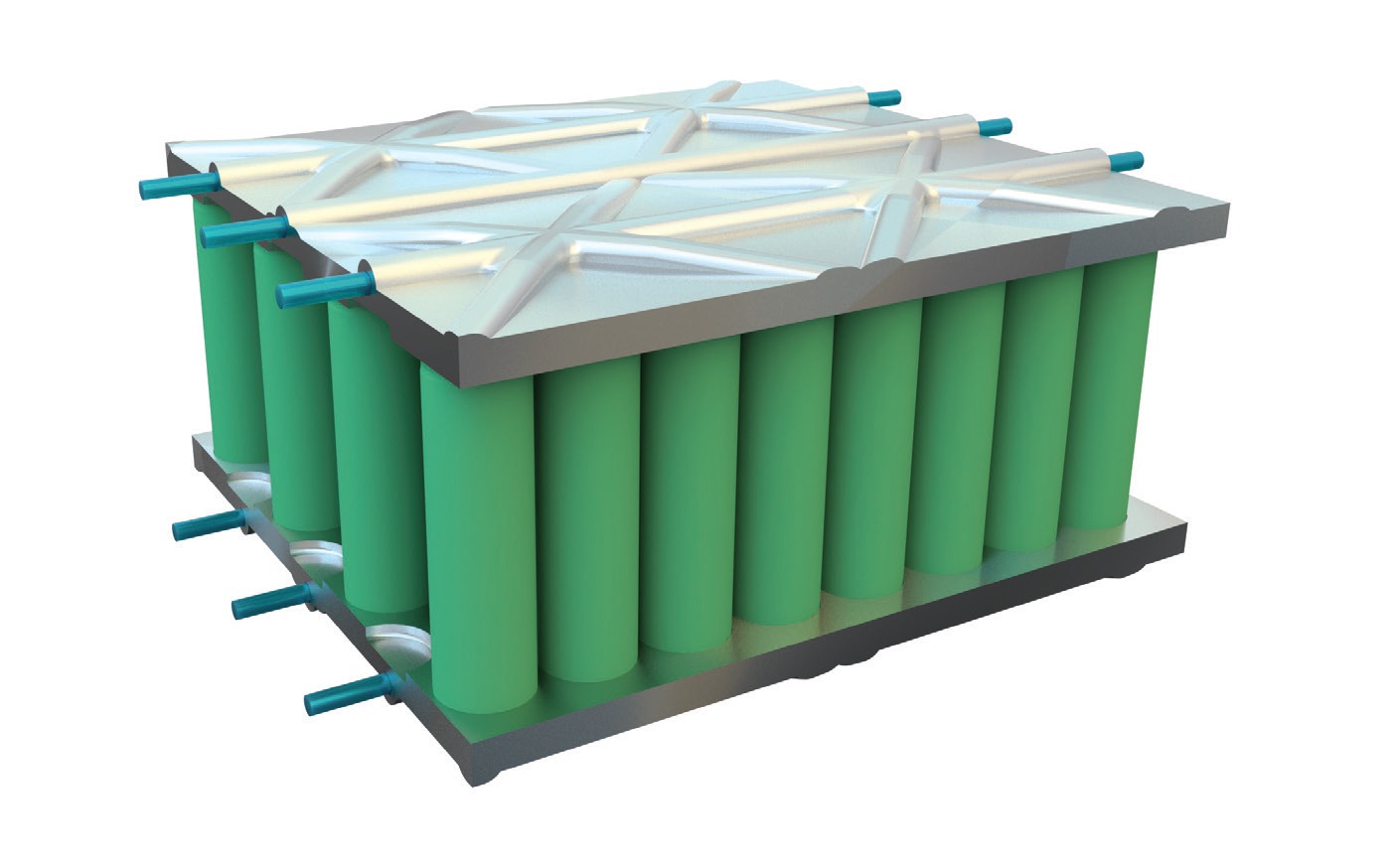
Figure 9. Another indirect cooling technique is known as bottom tray cooling. Figure courtesy of The Lubrizol Corp.
Sammut considers direct immersion cooling to provide several efficiency benefits, including improved safety, lower system cost and extended battery life. He says, “Immersion cooling can reduce the peak temperature of the battery and of bus bars. The latter are short strips of conductive metal used in high current electrical connections. This gives designers freedom to either downsize components, saving cost, or to extend the life of the battery pack.”
Sammut continues, “Better battery safety can be achieved because the direct immersion requires a dielectric fluid that will be less conductive and can prevent electrical injury if the fluid leaks or spills. Cost is reduced due to better efficiency and more precise/targeted cooling that does not need cooling jackets and leads to a reduction in the size and cost of battery pack systems.”
Sammut asserts that peak battery temperatures can drop by 8%-12% during battery charging. Additionally, battery pack temperature can be more uniform improving range estimations and reducing the likelihood of a cell failure.
Dr. Lotfi states that direct cooling has a unique advantage in providing a more uniform thermal management compared to the indirect method. He says, “Desirable electric properties are the key in enabling direct cooling. This is where hydrocarbon fluids provide an advantage compared to the water-glycol fluids used in indirect cooling.”
Testing
Alonso believes that the chemical industry is in the early phase of developing fluids for immersion cooling for EVs, and no industrywide tests have been established. He says, “The fluid must have the correct electrical properties (conductivity and dielectric properties), fluid compatibility with component materials, high thermal conductivity and a low freezing point (<-40 C), and reasonable viscosity or operating conditions will be required. Good performance in the battery nail penetration test will probably be required, and the fluid must have acceptable environmental, safety and health properties.”
Besides studying basic properties (such as viscosity, density, thermal conductivity and heat capacity) of heat transfer fluids, Dr. Lotfi feels that viscosity plays a critical role in thermal efficiency. He says, “Lower viscosity can improve thermal efficiency as it improves heat transfer and reduces pump resistance within the system. The best approach to evaluate the effectiveness of a heat transfer fluid is in an actual system (such as an e-motor and battery) as design parameters also can impact thermal efficiency within the system.”
Sammut points out that safety testing is important to evaluate the effectiveness of a heat transfer fluid in providing direct cooling. He says, “If in the event of a single battery cell failure, understanding how the fluid behaves and working to prevent a comlet battery pack failure is essential.”
Warren indicates that physical characteristics such as volatility, flash point, pour point, material compatibility and oxidation stability must be evaluated. She says, “Lower volatility means evaporation losses are lower and the cooling system does not have to be topped up as frequently. A higher flash point means better safety under high-temperature conditions. The fluid must have a low pour point so as not to freeze or become too viscous to pump at low temperatures. Materials within the cooling system such as elastomer seals, copper, insulating materials or any materials found within the cooling system must not be harmed by the heat transfer fluid. The heat transfer fluid must be oxidatively stable under elevated temperatures to the point that it may be expected to perform over the lifetime of the vehicle.”
Two other characteristics to be evaluated are electrical conductivity and breakdown voltage. Warren says, “The fluid must have limited electrical conductivity, to prevent arcing within the motor and/or power electronics. A high-performance coolant suitable for use in contact with energized components will have measured conductivity in the range of picosiemens per centimeter. Breakdown voltage, defined as the minimum voltage required to get current to pass through the fluid, should be as high as possible. Desired values should be in the 40-80 kilovolts (kV) range, if not higher.”
Low and stable electrical conductivity properties are important for heat transfer fluids not just in EVs but in other electronics cooling applications such as fuel cells. As an illustration, Table 1 shows data produced in the evaluation of an inhibited ethylene glycol/water-based heat transfer fluid. Mohapatra says, “This particular fluid exhibits stable electrical conductivity for 5,000 hours when maintained at 80 C.”
Table 1. An inhibited ethylene glycol/water-based heat transfer fluid displayed stable electrical conductivity (in microsiemens per centimeter) over a 5,000 hour period at 80 C. Table courtesy of Dynalene.
Challenges to commercialize immersion cooling
The importance of dissipating heat generated by the battery, electric motor, transmission and power electronics has led to the push to commercialize immersion cooling, which is more efficient than indirect cooling. But challenges remain that will need to be overcome.
Warren says, “Developers of immersion cooling fluids will need to keep three main areas in mind before achieving commercialization. Fluid compatibility is the first issue specifically with the very low viscosity fluids desired for more efficient pumping. But fluids with low viscosity have a negative impact on seals and other polymers. The reason is that lower viscosity fluids, in general, have smaller molecules that are more likely to interact with other materials. EV manufactures will need to consider the careful selection of certain materials, such as elastomers, while fluid manufacturers will need to be mindful of the formulation blends to mitigate compatibility issues.”
Warren feels that the automotive industry must adopt standardized testing methodologies to facilitate the commercialization of immersion cooling. She says, “In contrast to the internal combustion engine, the drivetrain technology in EVs is not mature; the market is developing rapidly and different OEMs approach cooling challenges differently—with competing designs. While there are industry established specifications for current engine oils or transmission fluids, no such standards exist for coolants. This creates additional challenges for fluid ingredient suppliers; without standardized assessment methods, we must work closely with OEMs and suppliers to understand their specific needs and design with them, fluids that suit their exact performance requirements.”
For the future, Warren predicts that immersion cooling will be a niche solution, at least for the next five years. She says, “We see total immersion cooling as part of the package for high performance, higher priced EVs. The presence of this technology may prompt the EV market to start to splinter into different categories—like we have now. For example, automobiles used in urban environments tend to have smaller engines and lower performance than larger vehicles. Smaller automobiles with smaller batteries and cheaper battery technology will be utilized in low-cost vehicles for shorter, urban journeys. EVs for cross-country, longer distances will need to have higher performing batteries that can be charged rapidly during short breaks in driving. Immersion cooling, with its engineering challenges, may be adopted by manufacturers creating high-cost, high-performance, long-range vehicles, where customers are happy to pay the price for the fastest charging. The result is that consumers will be faced with a cost versus need trade-off, where cheaper, smaller vehicles will probably continue with base plate cooling. Immersion cooling will remain as a secondary option until the EV market matures with growing production driving down the prices to the level of internal combustion engine-powered vehicles.”
Dr. Lotfi believes that selection of the proper base oil is critical in the development of a suitable fluid that can be used in immersion cooling. He says, “There is a trend for EVs to move to lower viscosity fluids to increase energy efficiency and thermal management in the drive units. Balancing low viscosity with other base oil properties such as volatility, flash point and tribological properties is critical to optimize performance and safety over the lifetime of EV fluids. Improved thermal conductivity and heat capacity can be realized with synthetic base oils compared to Group I, II and III base oils. Novel synthetic base oils with excellent balance of low viscosity and volatility and superior tribological properties can further enhance future technology advancement in EVs. They can help to push boundaries in EV fluid development and maximize performance in EVs.”
Heat transfer fluids perform important functions in industrial applications, and their use has expanded into renewable energy sources such as solar power. Concern about the significant heat generated during the operation of EVs has placed heat transfer fluids into a newer role where their use will be critical to optimize performance and efficiency. Immersion cooling has emerged as a new approach to enhance EV development and commercialization. The use of this technique will further highlight the important role that heat transfer fluids play now and in the future.
REFERENCE
1.
Please click
here.
Neil Canter heads his own consulting company, Chemical Solutions, in Willow Grove, Pa. You can reach him at neilcanter@comcast.net.