Rapid testing of tribotronic materials
Drs. Wilfred T. Tysoe & Nicholas D. Spencer | TLT Cutting Edge October 2021
Monitoring friction using a quartz crystal microbalance provides a rapid and convenient method for characterizing potential tribo-responsive materials.
The conventional philosophy behind designing lubricants for a particular application is to try to optimize them to function over the whole range of operating parameters so that, while they are not ideal for any particular situation, they work fine under all conditions. The challenge of achieving this is exacerbated as the machine ages and the conditions vary.
A solution to this problem would be to design lubricants with properties that can be changed by an external stimulus. They also could be integrated with condition monitors and placed under computer control, perhaps even integrated with machine learning, to allow the lubricant properties to be continually optimized. Such is the vision of “tribotronics,” which combines electronics and tribology to develop machines that can adapt to the operating conditions.
One possible method for rapidly and conveniently changing the friction of a lubricant would be to apply a voltage, thereby imposing an electric field on the lubricant that could polarize one component of the lubricant, possibly even inducing it to adsorb on the surface and modify the frictional behavior. It turns out that charged nanoparticles and ionic liquids can provide this tunability as different charged components adsorb on the surface as a function of the magnitude and polarity of the imposed voltage.
Unfortunately, it is difficult to design tribometers to rapidly and accurately measure friction while, at the same time, reproducibly imposing an electric field a cross the contact without the field influencing the measurement.
This problem has been solved by STLE member Jacqueline Krim and her graduate students, Caitlin Seed and Biplav Acharya, from North Carolina State University, by using a quartz crystal microbalance (QCM) to measure friction
(see Figure 1). Quartz crystals have a sharply defined resonant frequency f characterized by a large quality factor Q. The precise frequency has been used to accurately regulate clocks and watches. Since it depends on the oscillator mass, QCMs also have been used as precise balances, hence the name quartz crystal microbalance. However, because the outermost surface of the quartz moves laterally, the frequency also depends on the friction between the crystal and whatever is in contact with it. Because the quartz crystal is flat, it is easy to include a counter electrode to precisely apply a voltage to induce an electric field perpendicular to the surfaces
(see Figure 1, left).
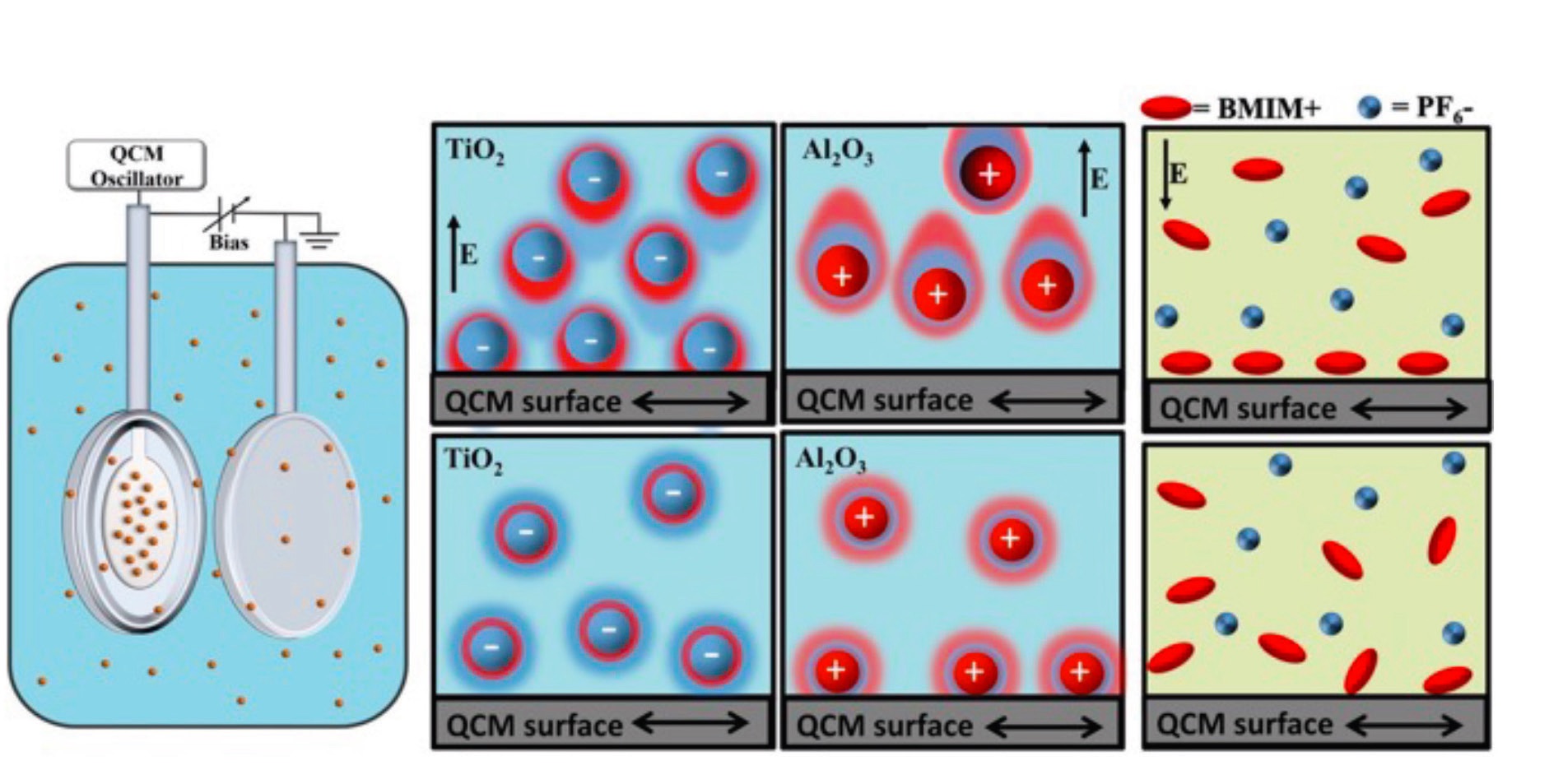
Figure 1. Schematic (left) of the quartz crystal microbalance (QCM) apparatus in the nanoparticle suspension, with a parallel counter electrode. Schematic (center) comparing TiO2 and Al2O3 nanoparticle movement under positive (top) and zero electric field conditions (bottom). Schematic (right) of ionic liquid movement under negative (top) and zero electric field conditions (bottom). Published with permission from Ref. 1.
This strategy was used to measure the change in friction as a function of bias voltage for an ionic liquid, 1-butyl-3-methylimidazolium hexafluorophosphate
(BMIM+ PF6‒, see Figure 1, right). The frequency shifts were measured with an alternating voltage, and the results are shown in Figure 2a. The frequency shifts indicate that the voltage can tune the friction and also provide information on the dynamics of the process by measuring the response as a function of the voltage frequency.
The group also explored the influence of nanoparticle suspensions, where the alumina nanoparticles are positively charged while the titania ones are negative
(see Figure 1, center). As demonstrated in Figures 2d and 2e, this predicts that alumina particles should be attracted to a surface with a negative bias, while titania should be attracted by a positive one. Figures 2b and 2c show the QCM frequency shifts as a function of the bias voltage, demonstrating the expected friction responses.
Figure 2. Left Panel: (a) Shifts in frequency for the ionic liquid BMIM+ PF6‒ at high (red circles) and low (black squares) frequencies. The response is greater in magnitude for lower frequencies. Right panel: Shifts in frequency (top) as a varying field is applied (bottom) to a 0.67 wt% Al2O3 nanoparticle suspension (b and d) and a 0.67 wt% TiO2 nanoparticle suspension (c and e) versus water. Published with permission from Ref. 1.
While the widespread application of tribotronics is still a dream, the use of rapid and convenient QCM friction measurements to screen the effectiveness and response times of a wide range of possible electric-field-sensitive materials will accelerate the development of this technology.
REFERENCE
1.
Seed, C.M., Acharya, B. and Krim, J. (2021), “QCM study of tribotronic control in ionic liquids and nanoparticle suspensions,” Tribology Letters,
69, p. 83.
Eddy Tysoe is a distinguished professor of physical chemistry at the University of Wisconsin-Milwaukee. You can reach him at wtt@uwm.edu.
Nic Spencer is emeritus professor of surface science and technology at the ETH Zurich, Switzerland, and editor-in-chief of STLE-affiliated Tribology Letters journal. You can reach him at nspencer@ethz.ch.