Hybrid additive manufacturing in the factory of the future
Lynne Peskoe-Yang, Contributing Editor | TLT Lubrication Fundamentals June 2021
Balancing additive and subtractive manufacturing might be the key to new efficiencies.
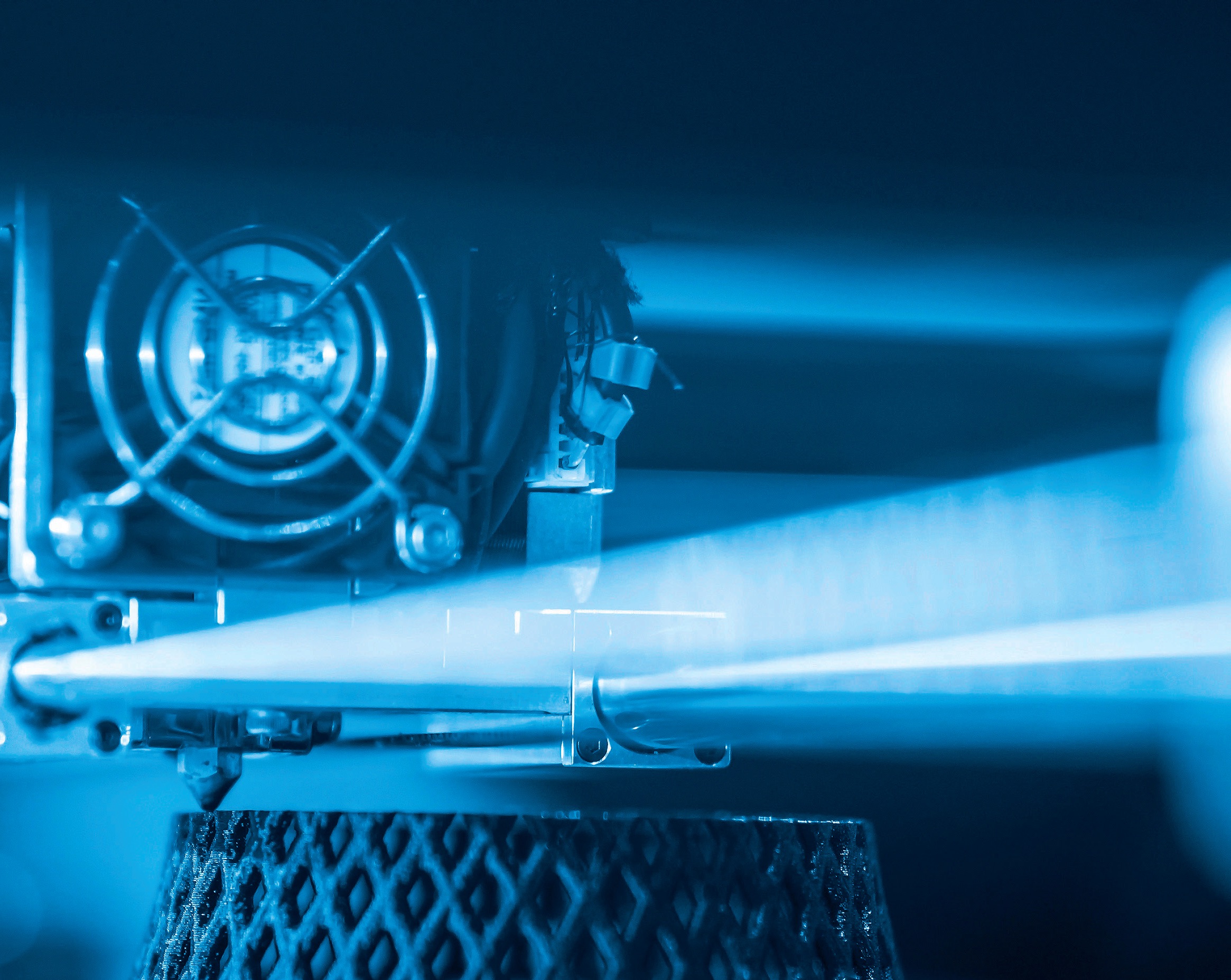
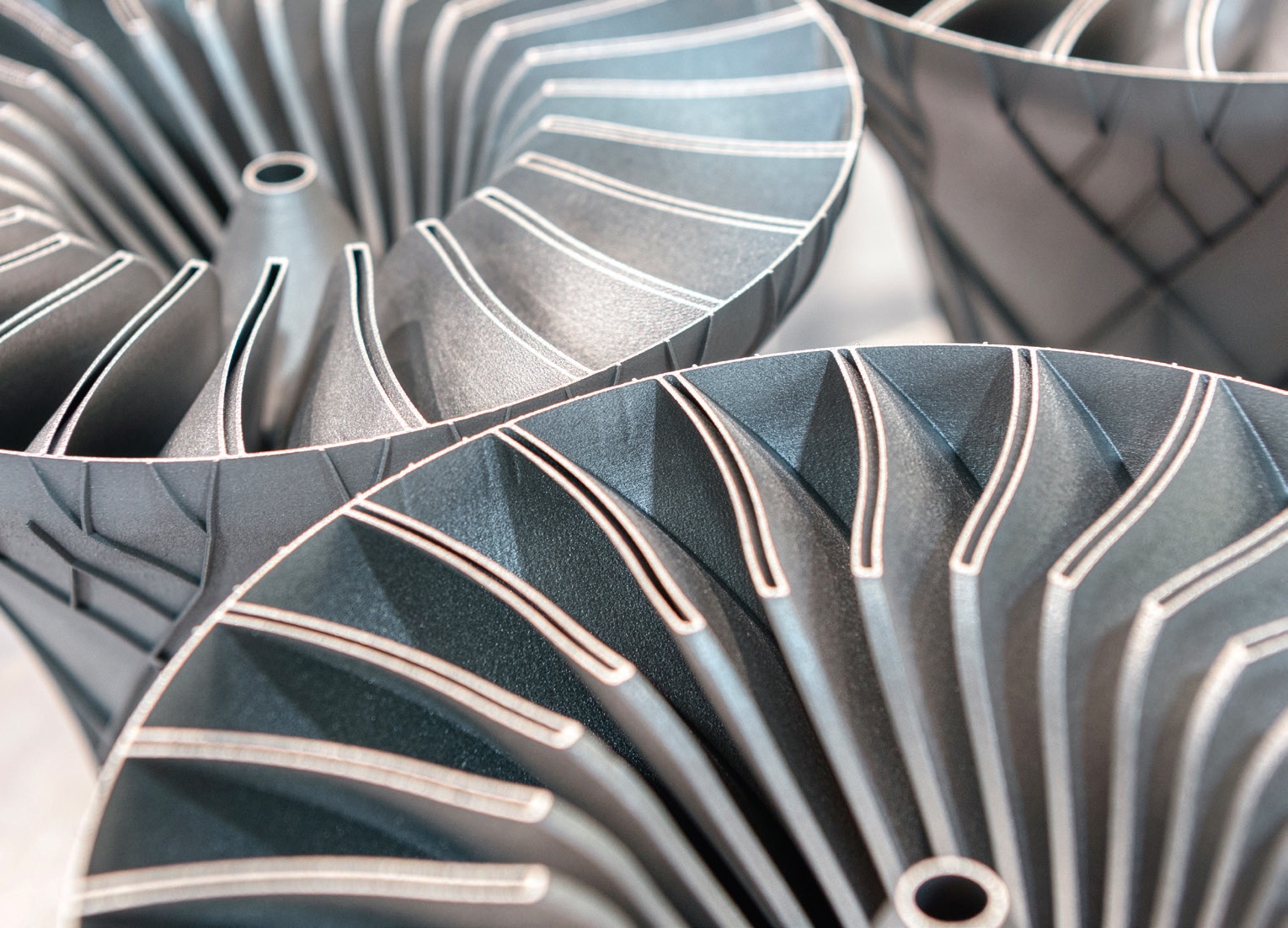
KEY CONCEPTS
•
Hybrid additive manufacturing combines additive and subtractive approaches to metalworking.
•
Common metal 3D printing techniques include the layered deposition and sintering of metal powders by high-energy beams.
•
Hybrid additive manufacturing enables new production efficiencies and greater complexity in surface engineering.
Computer numerical control, or CNC, is the industrial era’s response to an ancient legacy of subtractive manufacturing. While prehistoric sculptors chipped away at solid stone to reveal the figures of their imaginations, modern machinists use CNC to carve bulk metal into uniform parts with mathematical precision.
Additive manufacturing, also called 3D printing, takes the opposite approach, building parts from the inside out by adding raw material in successive layers. The technique has distinct economic advantages over subtractive manufacturing, as it minimizes waste and creates complex geometric forms without the introduction of new pressure points from fusing machined parts together.
But additive manufacturing has only recently been applied to the kinds of materials that would interest a machinist, and the fit is still not ideal. Despite its flexibility, 3D printing is not yet capable of producing metal surfaces with sufficient smoothness. “People talk about metal 3D printing as if what comes out of the printer is finished, and that’s rarely the case,” says Dr. Jason Jones, co-founder and CEO of Hybrid Manufacturing Technologies.
Jones was first hired into a 3D printing environment with the expectation that his subtractive experience would help to address the remaining deficiencies in 3D metal printing. “They had a CNC machine and said, ‘Do you think there’s any way we can combine these techniques?’” he recalls. “It seemed clear to me that these techniques are complementary—they’re not normally in opposition to one another. They’re very good at different things.”
From polymers to powders
While 3D printing, or “rapid prototyping,” has existed since the early 1980s, “the focus has predominantly been plastics,” explains STLE member Mandi McElwain, lubricant specialist at Univar Solutions. Early 3D printing processes were developed for photopolymers, which harden when exposed to UV light. Selective laser sintering, invented in 1989, achieved a similar feat on a metal substrate by training a laser beam on deposited metal powders to carefully fuse them into a continuous surface layer. Additional metal layers could then be added with more metal powders before the final layer solidifies.
Since then, the options for metal 3D printing processes have continued to expand. Dr. Wojciech Misiolek, chair of the Department of Materials Science and Engineering at Lehigh University, says the majority of techniques are still based on powders. “Powders are melted by lasers, or mixed with binders, and then the binders are removed and the metal sintered. There are techniques where powders are injected into the plasma flame and deposited into the substrate,” says Misiolek.
The hybrid approach
Once the layers are deposited, however, the metal part is subject to some of the same limitations as plastic 3D-printed parts: the surface isn’t finished. For advocates of hybrid manufacturing, this is where the CNC machining aspect truly shines. “At the end of the day, most of the time you don’t get the surface finish that you would desire, so there’s still going to be a need for CNC machining,” says McElwain. “That’s why hybrid manufacturing is so unique.”
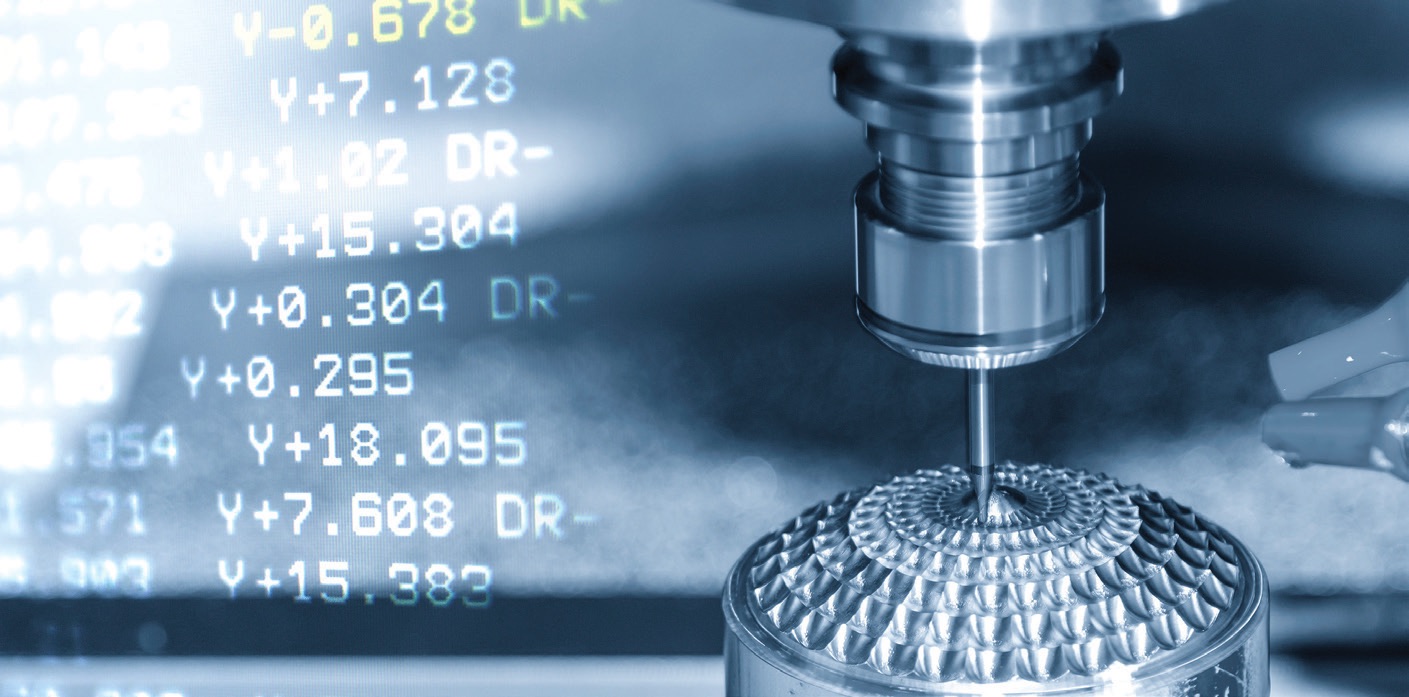
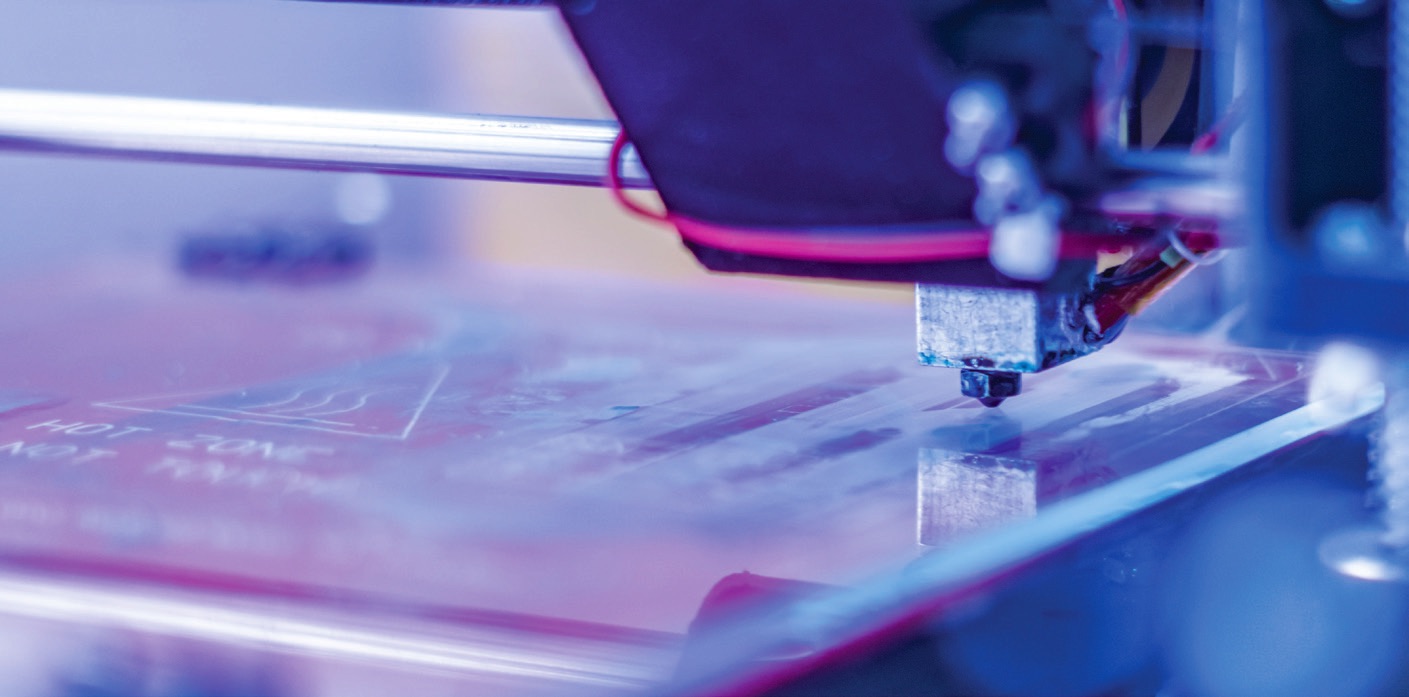
Combining additive and subtractive manufacturing works best with a customized production plan that reflects the strengths and weaknesses of each approach.
“Surface roughness and surface texture are highly important to how long parts last, but as-printed 3D printed parts are about as rough as sandpaper on average,” notes Jones. “How do you make them smooth?”
Hybrid manufacturing answers that question by intentionally intermingling additive and subtractive manufacturing processes, coupling the efficiency and speed of 3D printing with the surface control of CNC machining.
With its variable heads for machining, depositing and inspection, the combined setup can check its own work against predetermined specs before it moves on to the next steps and machine away any errors.
“It really closes a lot of the open loops that individuals otherwise have to track, maintain and correlate,” adds Jones. “You could almost think about it as the factory of the future—but in a single solution, a single setup.”
The hybrid advantage
Combining additive and subtractive manufacturing works best with a customized production plan that reflects the strengths and weaknesses of each approach. For all the precision and flexibility of CNC machining, there are a few tasks that are inherently easier from an additive approach. Smooth metal surfaces might be out of reach for 3D printing, but internal complexity is the perfect challenge: additive approaches can build intricate geometric structures, even in metal.
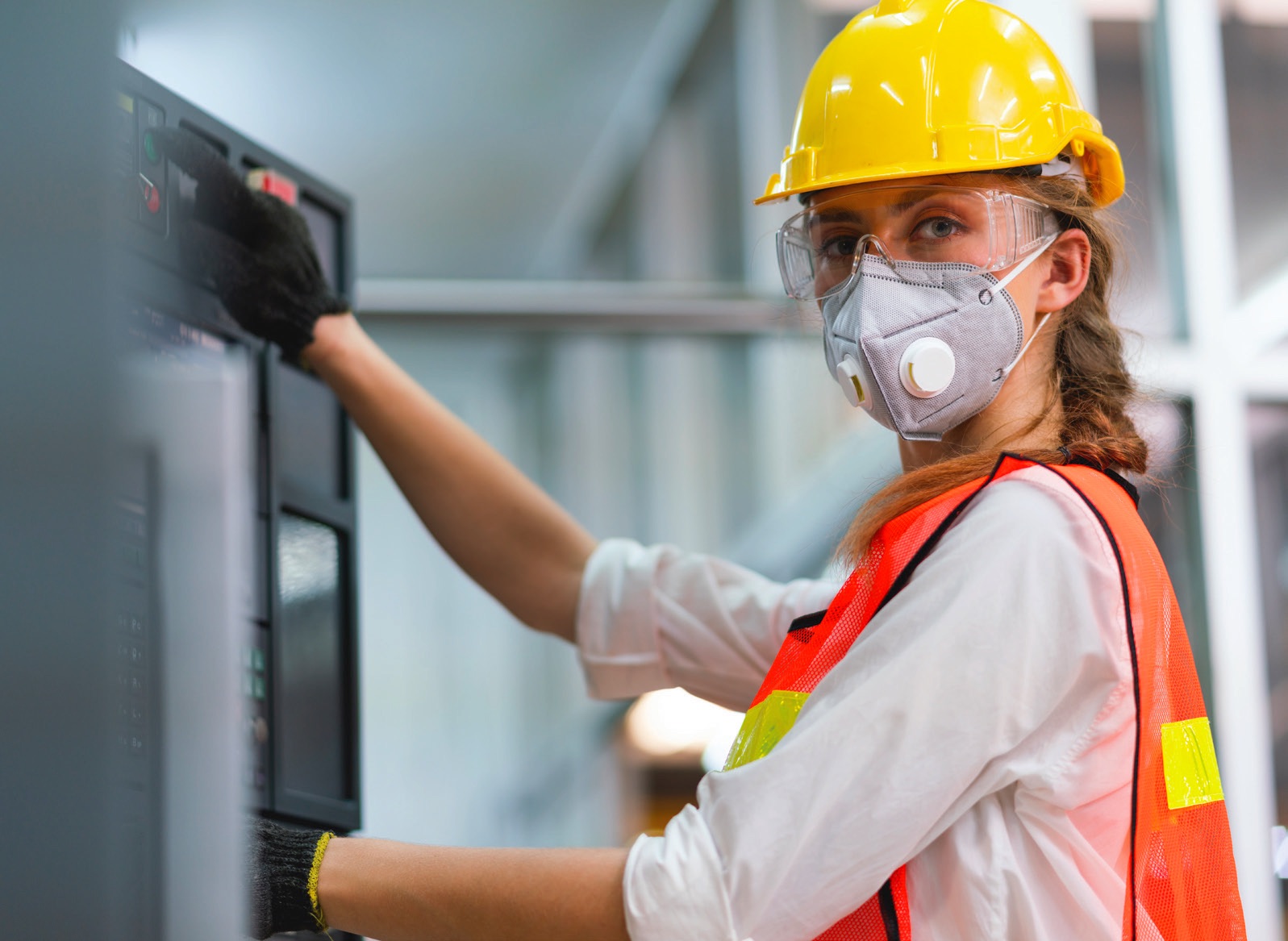
Hybrid setups might be transformative, but the transition doesn’t need to be painful. It helps that the geometric code, or G-code, which communicates the target area to the machine with three-dimensional coordinates, is consistent between additive and subtractive approaches.
“Casting or drawing or stamping doesn’t really afford the complexity of structure that you can achieve with a 3D-printed material,” says McElwain. “You really can’t get designs like pores or channels in one piece today. You’d have to weld them, which creates a point of stress in a high-temperature environment.” By producing these delicate designs additively and in a continuous fashion, 3D metal printing minimizes the risk of thermal stress points. This is especially useful for applications like tool manufacturing, where the raw materials are frequently classed as “hard to weld.”
Quality assurance in situ
Quality assurance is another area where hybrid manufacturing setups get extra mileage out of their dual approach. “With subtractive manufacturing, the final quality assurance steps usually happen at the end of the production line, and defective parts have to be reworked or discarded,” Jones says. In additive manufacturing, however, quality assurance is becoming an ongoing process: the 3D printer uses special inspection heads to test the integrity of every element. “With hybrid solutions, you can assess the quality in situ,” Jones explains. “If you find a defect or an anomaly, you can mill it away and add back on a new surface coating.”
In situ inspections can result in downstream efficiencies, as resources won’t be wasted on a part with an unfixable flaw, as well as valuable data for the redesign phase. “Maybe you need to adjust a parameter. Maybe you need a different material,” Jones says. “You’re able to do that all in a single setup and get the feedback immediately [to] the person who’s actually making the parts.”
Machining also lends its advantages to the hybrid setup. In addition to its superior ability to produce smooth metal surfaces, machining is the more efficient choice for producing certain kinds of part geometries, like those that have been designed for machining. Additive printers tend to be much slower, but that delay can be offset by a hybrid setup, which circumvents more expensive and time-intensive steps like shipping between suppliers, shops, inspection services and customers.
How shops are adapting to hybrid setups
Hybrid setups might be transformative, but the transition doesn’t need to be painful. “Within a couple of hours, any machinist can learn how to deposit metal, carbon fiber or polymers,” says Jones. It helps that the geometric code, or G-code, which communicates the target area to the machine with three-dimensional coordinates, is consistent between additive and subtractive approaches. “Adding additive capability to an existing CNC means it is the same machine they’ve been using, the same G-code, with just a couple of customized edits to accommodate additive techniques,” says Jones.
Operating a CNC machine is the perfect training for many aspects of 3D printing, with a few additions needed. Those who have worked exclusively in all-subtractive environments will have to get accustomed to answering for the inside and outside quality of materials they are now building by additive. “If you’re not used to adding anything, often you’re buying in a billet, which comes from a steel mill. You assume it’s solid metal, and because of routine high quality standards, you don’t worry about if there’s defects inside, such as discontinuities, porosity or other flaws,” explains Jones. “However, when you add the metal, you are now responsible for the outside and inside properties as well.
Adoption often starts with a shop printing its own fixtures, patterns and other types of manufacturing or assemble aids from polymers or composites. The first move into metal printing is often adding a small amount of metal to an existing part (such as a coating or adding a feature). This move allows one to get into the rhythm of production without the need to make significant design changes immediately. Ultimately the most effective use of additive manufacturing comes when paired with new designs. Jones advocates that this is the optimal application for hybrid manufacturing. However, readers are advised not to underestimate this step because as you start to design and build new complicated structures from scratch, managing the thermal gradient and resulting residual stresses can be tricky without robust simulation tools. Furthermore, the machining step can relieve some of that stress, which can result in distortion if not properly managed.
Designing for hybrid additive manufacturing
Purely subtractive manufacturing is well-suited to industrial production, where metal substrate can be purchased in bulk and uniformly machined, according to a common design. But the market for metal parts is changing significantly. End-users increasingly prefer custom parts in small lot sizes, which creates waste and reduces profit margins for established manufacturing methods like machining.1
Many applications for hybrid manufacturing are for surface engineering. Jones explains that often edges are reinforced or surfaces clad to increase wear resistance and thermal performance or to avoid corrosion and oxidation. This allows the use of lower-cost substrates and only requires the high-performing material where it is needed most. This opens up new opportunities for many applications including bearings, camshafts, bearing seats, gears, wear surfaces, molds, dies and high-temperature parts. The most popular materials to add are often difficult-to-machine alloys, including tool steels, nickel- and cobalt-based materials, and metal matrix composites.
This topic is taken one step further by Misiolek, who says that the most interesting questions remaining are about how various materials respond to the 3D printing process. “We are running a lot of tests, wear tests, looking into how long these materials are going to last at elevated temperatures,” says Misiolek. Some metals that would make great contenders for part production are difficult to render in powder form. Even materials that seem perfectly suited to the printing setup can run into technical issues in combination with other layers of the finished part. “The question is, ‘Can we do it right in the physics, in terms of getting the thermal expansion coefficients close enough so that they can work together?’” says Misiolek.
REFERENCE
1. Merklein, M., Junker, D., Schaub, A. and Neubauer, F. (2016), “Hybrid additive manufacturing technologies – An analysis regarding potentials and applications,” Physics Procedia, 83, pp. 549-559, click here.
Lynne Peskoe-Yang is a freelance writer based in Tarrytown, N.Y. You can contact her at lynnepeskoeyang@gmail.com.