Meet the Presenter
This article is based on a Webinar presented by STLE Education on July 22, 2020. Gas Engine Oil for Biogas Applications is available at www.stle.org: $39 to STLE members, $59 for all others.
Peter Harteveld has 40 years of industry experience and has been senior technical services advisor for Petro-Canada Lubricants, a HollyFrontier business, since 2007. He provides technical support for the oil and gas industry in Western Canada and supports Petro-Canada distributors in Latin America. He advises Petro-Canada customers about lubricant-related questions, visits job sites, inspects equipment and provides training. His areas of expertise include natural gas engines in oil and gas production, natural gas reciprocating and screw compressors, diesel and gas engines in power generation, steam and gas turbines in power generation, marine propulsion and auxiliary engines and heat transfer fluids and systems.
You can reach Harteveld at peter.harteveld@hollyfrontier.com.
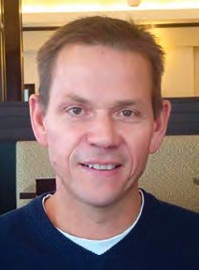
Peter Harteveld
KEY CONCEPTS
•
Biogases are a valuable and increasingly used energy source but pose challenges for engine components and gas engine oils.
•
Contaminants present in biogas can be removed prior to use to limit their adverse effects on lubricating oil performance and service life, as well as on engine component life.
•
Choosing the correct stationary gas engine oil (SGEO) is critical to operating biogas engines effectively for the long term.
Engines can be designed to burn gas (i.e., natural gas, biogas), gasoline or diesel fuels. Gas engines can be spark ignited, pilot diesel ignited or dual fuel engines that can burn both liquid and gaseous fuel. They operate at a constant speed and at a high, low or fluctuating load.
There is a near infinite variety of gas compositions possible in both natural gas and biogas, in contrast to liquid fuels, which are more limited in composition based on their octane rating or sulfur content. The gas composition strongly influences the stationary gas engine oil (SGEO) selected for a particular engine.
Gas burns more cleanly than most liquid fuels, but gas also burns hot and dry as opposed to liquid fuels, which results in higher lubricating oil stress in gas engines. The liquid fuel itself contributes to the protection of the valves inside the cylinder head of the engine, while gas fuel does not protect the valves. This means that the lubricating oil and its ash are required to protect the intake and exhaust valves in a gas engine. While some ash is needed, too much ash becomes a problem, as it can build up inside the combustion chamber, leading to cylinder liner wear, valve problems, pre-ignition and detonation (i.e., knocking).
Evolving engine design has resulted in greater horsepower output with increased combustion temperatures, resulting in higher local lubricating oil temperatures inside the engine. The oil has to be capable of handling the high temperatures. Modern engines are designed for better fuel economy, stricter emissions and smaller size to power ratios. Smaller engines mean smaller oil volumes, which translates to increased stress on the lubricating oil. Increased stress means higher degradation rates and shorter oil service life. Higher Brake Mean Effective Pressure (BMEP) and compression ratios make modern engines more sensitive to ash buildup, which can lead to pre-ignition and detonation.
The origins of biogas
Biogas comes from the anaerobic digestion or fermentation of organic material with the sources of material varying from manure, sewage, municipal waste, green waste and plant material. Table 1 summarizes the components of biogas.
Table 1. Biogas Composition
Figure 1 shows a comparison between natural gas and biogas. Natural gas contains a range of hydrocarbon components while biogas does not. Another difference is that biogas potentially contains contaminants, as summarized in Table 1. These contaminants influence the type of lubricating oil chosen for the biogas engine.
Figure 1. Natural gas and biogas comparison.
Impacts of contaminants using biogas as fuel
Biogas has a much lower combustion value than natural gas: 500-700 Btu/ft
3 for biogas, compared to 900-1100 Btu/ft
3 for natural gas (
see Figure 1). This difference is important because, to generate the same amount of horsepower, a biogas engine needs to burn nearly twice the volume of fuel gas as a natural gas engine, while the contaminants present in the biogas negatively impact the engine components and shorten the life of the lubricating oil.
When biogas is burned, hydrogen sulfide present in the gas is converted to sulfur dioxide and sulfuric acid, which contaminate the lubricating oil and shorten its service life. Engines that operate with frequent starts and stops, or operate under partial loads, experience more intense engine component corrosion from acid condensation. In addition, liquid water in the gas causes fouling, deposits and rust.
When the organosilicon compounds found in landfill gas and sewage gas are burned, they can form quartz sand inside the engine cylinders. These sand byproducts cause abrasive wear and add to the deposit buildup of lubricating oil ash (
see Figure 2). This ash can get loose and be ground down to a fine abrasive powder that wears down the engine surfaces. It also can cause valve torching, cylinder liner wear, pre-ignition, detonation and knocking.
Figure 2. Deposit buildup of lubricating oil ash and biogas contaminants. Figure courtesy of Petro-Canada Lubricants.
Chlorine contamination present in landfill gas leads to hydrochloric acid from combustion. Fluorine contamination in landfill gas leads to hydrofluoric acid. Ammonia contamination in biogas leads to nitric oxide and nitric acid. These acid and salt byproducts cause corrosion of engine components and shorten both engine component life and lubricating oil service life.
Hydrogen contamination is not desirable in a biogas because it interferes with flame travel. Combustion inside an engine is not an explosion. Instead, combustion is a controlled process of burning fuel with controlled propagation of the flame front inside the cylinder to produce hot expanding gases that drive the piston down in the power stroke. Hydrogen has a 10 times higher flame speed than methane and interferes with the flame front propagation. Hydrogen also increases flame temperature, which results in the formation of nitrogen oxides that further nitrate the lubricating oil.
The combustion value and composition of landfill gases can vary, depending on the types of waste in the landfill cell where the gases originated. Engines running landfill gas need a good air-to-fuel ratio controlling system to provide flexibility for those changes in fuel gas composition and combustion value.
Biogas treatments to remove contaminants
Biogas treatment removes these contaminants to make the gas as clean as possible, which is important for reliable engine operation. Desulfurization technologies are physical-chemical technologies, including ferric chloride dosing that precipitates hydrogen sulfide, or activated carbon filtration that adsorbs hydrogen sulfide. There are other technologies available, including biotechnologies, but they are not as effective (e.g., biofiltration using microbes, biological treatment with aerobic bacteria).
Removal of siloxanes relies on adsorption or adherence of the contaminants to a porous material (e.g., activated carbon, silica gel desiccant) in combination with refrigeration, regenerative adsorption, etc. The treatment technology selected depends on the intended use of the gas and the variability of the gas composition.
SGEO degradation modes
The SGEO used in gas engines degrades through three primary chemical mechanisms: oxidation, nitration and the effects of sulfur species present in the fuel gas—the latter expressed as sulfation. Oxidation and nitration of lubricating oil occur with both natural gas and biofuel, while sulfation occurs predominantly with biofuel.
Oxidation of the lubricating oil is a reaction of the base oil with oxygen, which results in increased oil acidity and carbon formation in the piston ring area, eventually inhibiting ring movement. Oxidation occurs in both ultra-lean and lean burn engines.
Nitration of lubricating oil is a reaction of the base oil with nitrogen increasing oil acidity and leading to the formation of sticky nitrated sludge, which is difficult to remove and shortens oil service life. Nitration occurs in rich burn (stoichiometric) engines.
Sulfation, also known as the sulfation level or sulfate index, is the formation of sulfur-based acids that react with additives and the base oil. Oil acidity increases, which shows up as a high acid number (AN) in a used oil analysis. The oil initial pH (ipH)—which indicates the strength of the acid—decreases, which increases the corrosion potential of the oil. When lubricating oil degrades as a result of the formation of sulfur-based acids, sludge and varnish form, viscosity increases, and sedimentation takes place. The chemical mechanism is a reaction between oxygen, water and sulfur in the fuel gas. The effects of sulfation can be aggravated by low oil temperature, which results in acid condensation and manifests as shortened oil life and corrosive wear of engine components as the oil becomes more acidic.
Ash importance
Lubricating oil ash protects the valves in a biogas engine. Ash is composed of inorganic noncombustible material present in the oil. Factors that influence ash formation in the engine combustion chamber include:
•
Additives like detergents, dispersants and antiwear components contribute to ash buildup in the combustion chamber of an engine. The amount of ash buildup varies, depending on the additive treat rate and the detergency/acid neutralization balance of the additive package.
•
The higher the engine’s rate of oil consumption, the higher the ash buildup will be.
•
Biogas composition can impact ash buildup, depending on the type of biogas being used.
•
Heavily loaded engines operating at 80%-100% load tend to stay cleaner than more lightly loaded engines. Engine models react differently to ash buildup, while how an engine is set up to operate also impacts ash formation.
How to select SGEO
Knowing the gas composition as it enters the engine is one of the factors used in the selection of the SGEO. The selection of the optimum lubricating oil depends initially on the OEM recommendation.
Other factors to consider in lubricating oil selection include the sulfated ash content of the oil in consideration with the fuel gas composition and quality, the load on the engine and the engine make, model and engine history. The most suitable product is selected based on a number of factors, including:
•
The desired oil service life (i.e., the maintenance schedule).
•
The acid neutralization capacity needed, or the required corrosion protection based on the mode of operation (i.e., peak shaving type of operation with lots of starts and stops providing cool down opportunities versus continuous operation at high load with no cool down).
•
The engine sensitivity to combustion chamber component deposit formation.
When it is necessary to switch lubricating oil, the user should rely on the used oil analysis trending of the in-service product to tell how an oil behaves under the specific conditions.
SGEO additive technologies
In general, SGEOs for biogas applications can be divided into four groups based on additive chemistry:
•
Low ash SGEOs for pretreated gas only. These lubricating oils are suitable for biogas service but are formulated for natural gas service. Predominately, these oils are SAE 40 with low ash content (<0.6%) and combine oxidative stability with nitration resistance but have a limited acid neutralization capacity. These oils can only be used for pretreated biogas applications where the biogas has low chlorine and ammonia content, the silicon content matches OEM specifications and the hydrogen sulfide content is less than 200 ppm.
•
Low ash SGEOs formulated for contaminated biogas applications. These lubricating oils are SAE 40 with low ash content (<0.6%) with an additive package that provides control over acid formation. The oils have advanced detergent/dispersant technology and contain corrosion inhibitors and metal passivators. The oil forms a protective film between the acids and the engine components and can be used in applications with moderate to high chlorine and ammonia content, silicon content according to OEM specifications, hydrogen sulfide greater than 200 ppm and high halogen gas.
•
Conventional mid-ash SGEOs formulated for contaminated biogas applications. These lubricating oils are SAE 40 with a moderate ash content (0.6%-1.0%). The oils contain more alkaline materials, so the base number is higher, correlating to a higher acid neutralization capacity. The higher neutralization capacity results in the oils neutralizing the acids rather than forming a protective film. These oils provide a longer oil service life as they have more additive to deplete. They can be used when the biogas has low to moderate chlorine content, moderate to high ammonia content, silicon content according to OEM specifications, more than 200 ppm hydrogen sulfide and low to moderate halogen gases.
•
Modern mid-ash SGEOs formulated for contaminated biogas applications. These lubricating oils are SAE 40 with a moderate ash content (0.6%-1.0%) and are formulations designed with advanced detergent/dispersant technology to provide acid neutralization and maintain cleanliness combined with corrosion inhibitors and metal passivators to protect engine components from corrosive wear. These types of lubricating oils should be used with highly contaminated biogas fuels.
There are tools available to help in selecting the appropriate SGEO, including the online SGEO selection tool (
see Figure 3) and the SGEO selection table available online
here.
Figure 3. The online SGEO selection tool available here. Figure courtesy of Petro-Canada Lubricants.
The consequences of selecting an unsuitable SGEO include short oil service life, corrosive wear of engine components, excessive combustion chamber deposit formation, cylinder liner wear, valve problems, high lubricating oil consumption, shorter oil filter service life, production loss and increased maintenance and labor costs. These consequences can jeopardize engine longevity, durability and reliability. Some of these consequences are illustrated in Figure 4, which shows corrosion, wear and damage of engine components.
In contrast, Figure 5 shows the positive effects of choosing the correct SGEO where the engine shown has operated for 24,000 hours with no negative impacts.
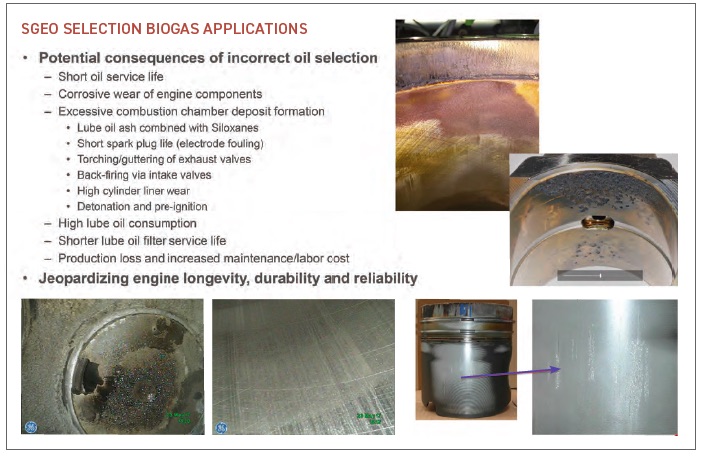
Figure 4. Consequences of selecting an unsuitable SGEO. Figure courtesy of Petro-Canada Lubricants.
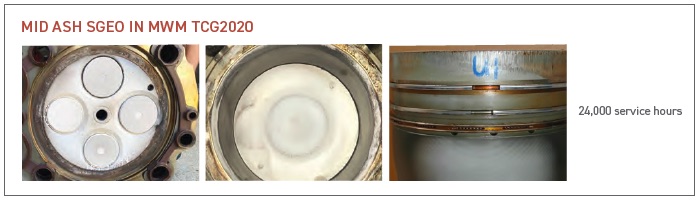
Figure 5. Positive effects of choosing the correct SGEO. Figure courtesy of Petro-Canada Lubricants.
Conclusions
As biogas is recognized as an increasingly valuable fuel source, tribology will play an important role in biogas use. While biogases can contain a variety of different contaminants, these can be removed prior to use to prevent negative impacts on engine operation and can be handled through appropriate SGEO selection. The correct SGEO is critical to operating biogas engines effectively and efficiently for the long term.
Andrea R. Aikin is a freelance science writer and editor based in the Denver area. You can contact her at pivoaiki@sprynet.com.