The rapidly expanding field of biotribology
Jeanna Van Rensselar, Senior Feature Writer | TLT Biotribology December 2020
Between the development of better medical devices, more responsive touchscreens and even more effective skin creams, biotribology has never been more important.
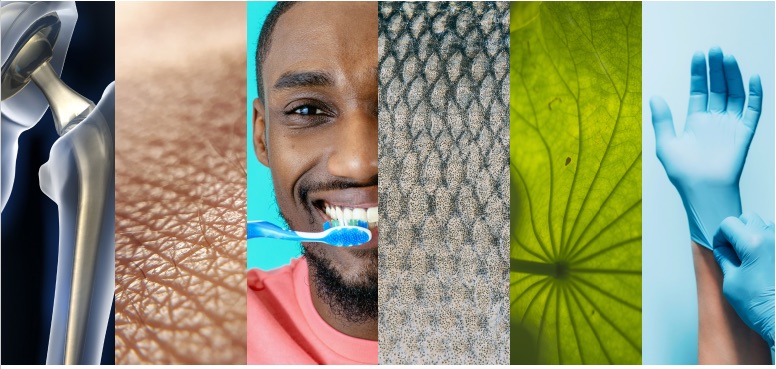
KEY CONCEPTS
• The basic classifications of biotribology include joint tribology, skin tribology, oral tribology, tribology of the other human bodies or tissues, animal tribology, plant tribology and medical devices. Some thin solid coatings form self-replenishing lubricant films and last for the lifetime of the devices they protect.
• One of the primary testing challenges for biotribology is the very limited opportunity to test biological materials in living beings. Other challenges include matching physiological conditions and maintaining homeostatic ranges.
• Increased collaboration between biotribology-related disciplines and industries will allow the field to advance rapidly.
Biotribology affects the lives of everyone every day whether they know it or not—from blisters to contact lenses, facial moisturizer, toothpaste and digital devices. Relatively recently, the development of touchscreen technology has highlighted the importance of haptic1 feedback driven by friction in the screen/finger interface. Research into these and many more areas furthers the understanding of lubrication and wear in general.
The relevancy and success of tribological research depends on the ability to conduct friction and wear measurements in the lab. However, biomaterials are complex with a range of unique thermomechanical properties that make testing a challenge.
Research is increasingly focused on obtaining a clearer tribological picture of the biological interface. Dr. Tannin Schmidt, associate professor, department of biomedical engineering, University of Connecticut Health Center, says, “I think it’s the growing understanding and recognition on how important a role friction can play in various biological systems. In that sense, an increased ability to measure and understand friction in a particular system can often lead to an increased understanding of that system itself—be it in health or disease—the latter of which can often contribute to the development of new drugs or products to help treat diseases or conditions. A perfect example of that is contact lenses, since reducing friction has actually shown to be correlated to reducing discomfort when people wear them.”
Assuming that biotribology testing equipment and testing demand are good indicators, Europe and North America seem to have a particularly strong interest in biotribology. Pierre Leroux, CEO of Nanovea Inc., says, “The demand we have seen is from larger corporations based in North America and Europe that are involved in biomedical devices, advanced cosmetics and the latest prosthetics.” Nanovea designs and manufactures tribosystems and other materials testing instruments.
STLE-member Angela Pitenis, assistant professor, materials department, University of California, Santa Barbara, explains that decades of research have been dedicated to the tribology of biological interfaces, from the human body (e.g., articular cartilage, skin, hair, eyes and mucosa) to the animal kingdom (e.g., snails, marine mussels, barnacles, geckos, click beetles and fossilized dinosaur teeth, see The Biotribology of Animals) and the natural world (e.g., lotus leaves, biofilms and biopolymers).
“The richness and complexity of biology calls for more sophisticated tribology approaches to interrogate biological interfaces with minimal interference or damage,” she says. “I think tribologists are drawn to impossible problems. Tribology research has touched space, ultra-high vacuum, ultra-low temperatures, supercomputers and, in a way, biology is the next frontier. Roughly 50 years ago, the late Duncan Dowson coined the term biotribology, and accurately predicted this emerging field would become increasingly important to human life and health.”2
Applications
The basic classifications of biotribology include joint tribology, skin tribology, oral tribology, tribology of the other human bodies or tissues, animal tribology, plant tribology and medical devices.3
• Joint tribology: hip and knee joints, joint fluid, joint restorative materials, articular cartilage, the interfaces of implants, etc.
• Skin tribology: perception induced by skin friction, skin friction and gripping of objects, skin care products, artificial skin, how skin interacts with articles such as shoes and socks, sports devices, cosmetic treatments, skin discomfort, irritation, etc. (see Figure 1 and The Biotribology of Skin).
• Oral tribology: saliva, tongue, natural and implant teeth, restorative dental materials, toothpaste, mandibular joints, swallowing, etc. (see Figure 2).
• Tribology of the other human bodies or tissues: anything related to capillary blood flow, bone cells, hair, contact lenses, ocular surfaces, etc.
• Animal tribology: animal movement, fish/shark skin, bird feathers, unique properties of certain animals such as gecko adhesion, etc. (see Figures 3, 4 and 5).
• Plant tribology: diatoms, unique properties of certain plants such as lotus leaves.
• Medical device tribology: artificial cardiovascular systems, medical gloves, scalpels, forceps, catheters, gastroscopes, etc.
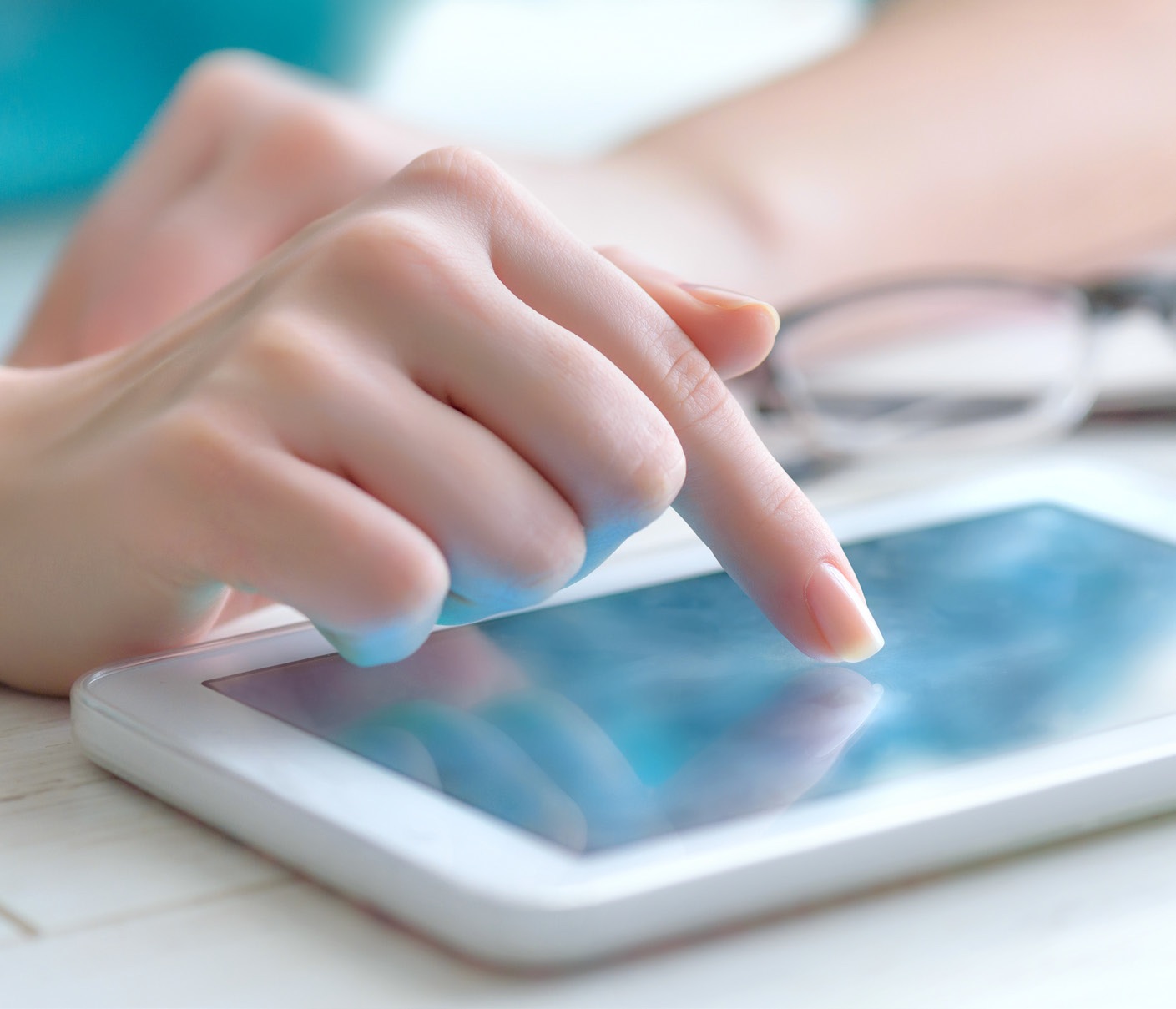
Testing and issues with testing
The field of biotribology is comprised of topics ranging from the application of cosmetics on human skin to studying complex interactions between invasive tubes and human tissue. Dr. Kartik Pondicherry, principal scientist – tribology for Anton Paar GmbH, explains, “In terms of developing new equipment, what drives us the most is the quest for finding novel solutions, especially when it comes to biological applications. For instance, when we think about cartilage or implants, we are actually talking about the possibility of improving the quality of human life.”
According to Pitenis, the practical challenges of any biotribology experiment are:
1. Obtaining samples for repeat experiments (acquiring biological samples might be difficult, expensive or might need special approvals)
2. Selecting appropriate test conditions and counterface materials, geometries and scales
3. Matching physiological conditions (e.g., contact pressures, shear stresses, sliding speeds)
4. In the case of living biological systems, maintaining homeostatic ranges (e.g., temperature, humidity, environmental gases) throughout the experiment.
“From a tribology perspective, biology is nearly the antithesis of conventional engineering systems,” Pitenis says. “Biological surfaces are highly irregular; organic materials are complex, heterogeneous and often fragile; and the body’s environment is typically aqueous, dynamic and aggressive.”
The samples themselves can be the primary issue for tribological measurement in biological systems. Schmidt explains, “In my experience, the challenge is usually the sample preparation and mounting. Working with biological tissues, be it cartilage, cornea or eyelids, it can be more difficult than typical engineering materials that can be cut, held and mounted easily. So, getting a (often precious) biological sample prepared and mounted for friction testing is often the most challenging, and most important, part.”
Most of the interfaces that Pondicherry observes can be categorized under large-area conformal contacts with relatively low contact pressure. “The first hurdle that we face is the availability of real-life specimens or appropriate surrogates for the same,” he says. “Then there is the issue of fixing the specimen, which, in some cases, is especially difficult when the samples are soft and fragile. However, the biggest challenge that I see is to be able to build a model to correlate tribological data from model-scale tests with behavior of the system in the real-life application. This would only be possible when we have access to data from the field, which is often not the case.”
He continues, “Obtaining appropriate substrates/sample materials is a challenge. Using substrates/samples that are actually used in real-life applications is the best option in most cases. However, this is easier said than done when dealing with biological applications. Using surrogates for biological samples from bovine or porcine sources is prevalent, but due to the inhomogeneity in the samples themselves, it is sometimes hard to discern the source of minute differences observed in the test data. Therefore, at times, use of nonbiological substitutes is the only option.”
Pondicherry explains that while it is easy to procure artificial skin, finding surrogates for cartilage or other biological tissues is not that simple. “In such cases, we do team up with experts from both academia and industry to find the most reliable and cost-effective way of obtaining appropriate test specimens,” he says. “For instance, a couple of years ago, we partnered with an international research group from Japan to gain knowhow into creating a hydrogel that we recommend to our customers for select biological applications to imitate certain human tissues.”
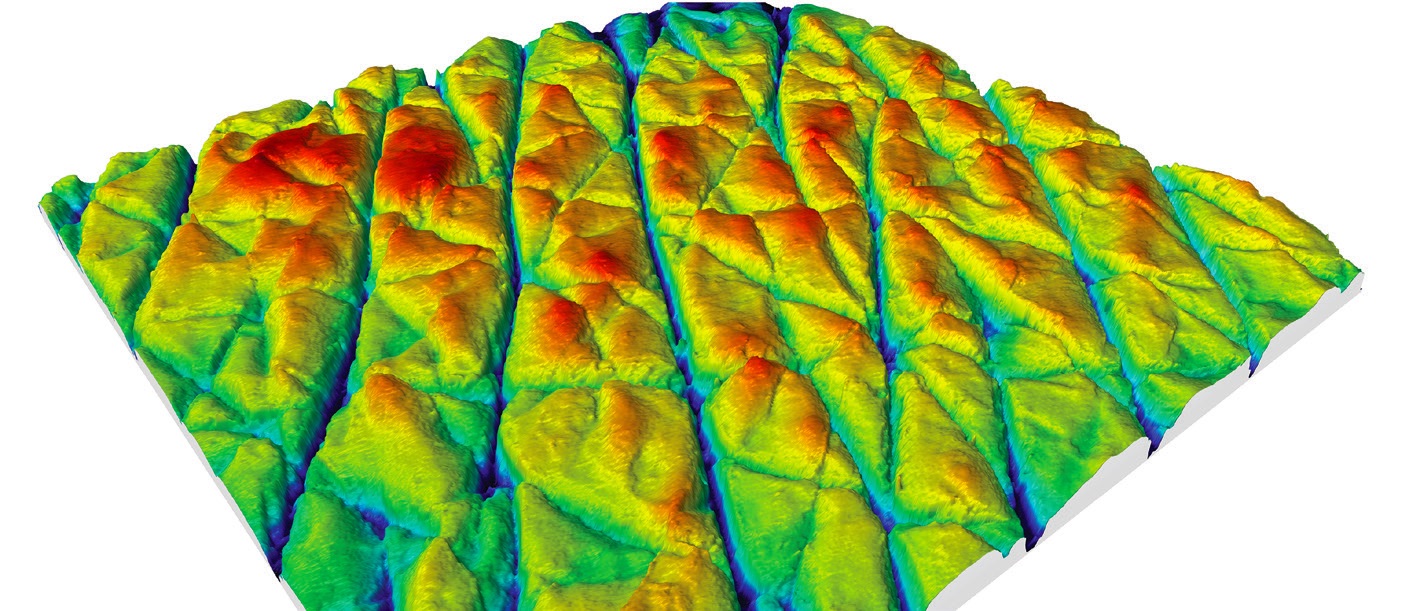
Figure 1. Human skin scan, obtained by Nanovea Labs using Nanovea Optical Profilers. Figure courtesy of Nanovea.
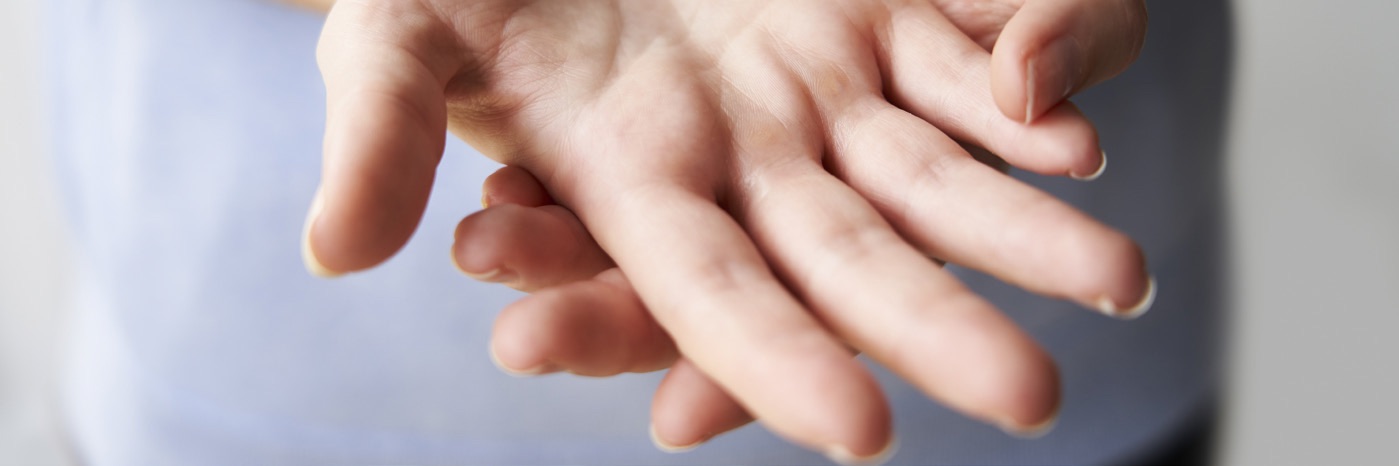
The biotribology of skin4
Skin tribology might be one of the most prevalent areas of biotribology research, and it presents major challenges, mainly because human skin is multifaceted and subject to many factors—both environmental (sun, temperature, humidity, pollution, cosmetic treatments) and personal (ethnicity, gender, age, health and diet).
Skin tribology is a good example of biotribology that affects everyone 24/7. And it’s not just about face creams and insect repellent. Much of skin tribology addresses how hands interact with objects: the degree and quality of the friction of palms and finger pads and how it informs such activities as gripping and manipulating objects.
The friction between the soles of feet and the contact surface is important, especially in dangerous situations such as wet or unstable flooring. Reducing friction between fabric and skin is a necessity for clothing comfort and avoidance of skin irritation/blisters. Interaction of skin and shaving razors and skin and medical devices such as face masks for sleep apnea are all predominantly affected by friction levels.
Because everyone’s skin is different, it is very difficult to generate data that is universally applicable (i.e., friction can vary greatly between different skins subject to the same nominal environmental and loading conditions). This means that test methodologies must be well controlled, rigorous and involve a wide range of participants to yield meaningful results.
Because of the testing challenges involving biological skin, another approach is to use a statistically comparable alternate material. The artificial skin materials that are currently available are not specifically designed for tribological performance and are usually used to train medical practitioners to perform procedures that involve cutting or piercing skin. Given this, researchers are working hard to find a scientifically acceptable skin replacement for tribological testing purposes.
The field of skin tribology covers an astonishing array of applications and industries, and much of the work in this area has significant commercial potential. Right now, there is a diversity of work underway on skin tribology in terms of measurement, imaging, modeling and understanding how skin protection, such as medical gloves, influences friction and feel.
Schmidt adds that another key challenge is that the friction coefficient is really a system variable. “For example, it can depend on test setup/variables, geometry, scale, counterface and, of course, lubricant,” he says. “This can lead to challenges, discussions and arguments when comparing data from different studies.”
He adds, “The choice of counterface can be especially important in biotribological studies (i.e., if one is measuring putative lubricants for cartilage, or lubricants for contact lenses, what is used as a counterface can greatly affect results). We published a paper5 looking at the effect of various cartilage lubricants present in our synovial fluid, keeping everything constant except to compare a cartilage-cartilage biointerface versus a cartilage-glass interface. What we found is that a lubricant can effectively reduce friction at one and not at another. This just reinforces the point about friction being a systems variable, and when trying to measure friction of biological tissues—just how important counterface and test setup can be. That should always be kept in mind when trying to interpret, compare or extrapolate in vitro results.”
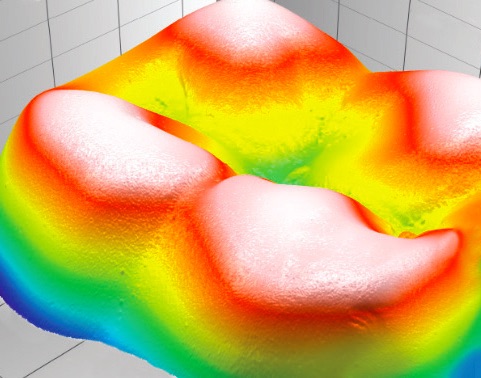
Figure 2. Tooth scan, obtained by Nanovea Labs using Nanovea Optical Profilers. Figure courtesy of Nanovea.
In vitro versus in vivo
One of the primary testing challenges for biotribology is the very limited opportunity to test biological materials in living beings. “Many research efforts in biotribology are initially focused on in vitro as opposed to in vivo measurements for several reasons, including convenience and consistency,” Pitenis says. “Unlike typical tribological systems, accessing buried biological interfaces in vivo might inflict pain or irreparable damage. For this reason, in vitro studies of biological materials (e.g., cells, cell layers, tissues) are performed outside of their natural context and often in labware like glass-bottomed culture dishes. This approach can reduce sample-to-sample variability and provide more uniform surfaces for friction measurements.”
Pitenis continues, “Although in vitro 2D and 2.5D measurements are useful and informative, the leap from bench to bedside often relies on in vivo and/or clinical studies. Several very talented groups have performed in vivo friction measurements against living biological interfaces, including the cornea of an anesthetized mouse and the skin of willing human participants.”
Schmidt adds, “I have often thought about if/how one could measure friction on a human eye—which I’m sure would be of great interest to various contact lens companies—but I think there is a long way to go in that respect.”
Biotribological test equipment
In terms of friction and wear in biotribology, there is a vast area of testing that requires very low pressure on a relatively large area. “There are many examples, but to give a few areas of study—hand skin moisturizer, pacemaker cabling inside the body, stent delivery system and toothpaste or flossing material,” Leroux says. “In some cases, like hand moisturizer, being able to study coefficient of friction is important to compare various formulations. In other cases, such as pacemakers, the possible repeated low pressure rubbing of cabling on bones can become a direct cause of a life-threatening failure.”
He continues, “The equipment used in biological testing applications has different requirements than testing equipment for industrial situations. “Many applications require very low surface pressure, and often a simulation of a liquid environment is critical to replicate real-life conditions.
Even at higher pressure applications, such as joint implants, simulating the natural lubrication is essential to understanding wear and friction.”
Issues
“Over the last few years, I have seen a growing enthusiasm from larger manufacturers to respond to the biotribology community’s needs and deliver tribological instrumentation suited for soft and biological materials research,” Pitenis says.
Schmidt says he has been able to measure friction of various tissues with commercially available instruments. “The limitations of the systems, I would say, come back to challenges associated with sample mounting,” he explains. “So, even if the instrument has the sensitivity and maneuverability, in terms of movement as well as measuring forces, custom development of sample holders is still often required.”
Pitenis adds, “Biological samples might span several orders of magnitude in mechanical and transport properties (for instance, bone versus brain tissue) and demand tailored instrumentation, including integrated imaging capabilities. I think improvements in commercial systems will increase accessibility and encourage more engineers, scientists and scholars to pursue studies in biotribology.”
Pondicherry thinks that some existing tribometers are capable of studying certain biological interfaces. “It all boils down to whether or not an instrument can accommodate the test specimen and set and measure the test parameters in their relevant ranges,” he says. “For instance, if you take the case of food tribology, the most commonly used instruments are actually slightly modified versions of existing devices.”
He continues, “Over the past decade, we have worked extensively toward developing test setups and methodologies for numerous biological applications, ranging from ocular systems to implants and biomedical devices. Moreover, at Anton Paar, we can invest a part of our time and resources toward research and developmental activities that we find interesting, without being concerned about the financial returns. One has to keep in mind that most of these developments are not yet commercially viable as we are dealing with a niche market here.”
Conclusions
Pondicherry would like to see more collaboration between biotribology-related disciplines and industries in the future. “In my opinion, the dearth in the dissemination of information is a huge impediment in the path to progress that one wishes to achieve,” he says. “However, over the past few years, we have seen a growing trend toward sharing of knowledge through various platforms, including dedicated conferences on biotribology and dedicated sessions on the same topic at important events such as the STLE Annual Meetings.”
He adds, “During my talks at conferences and workshops over the past years, I have been quite vocal about active collaboration between industry, academia and instrument manufacturers. This is especially true for emerging fields with a huge potential for advancement. So far, the best results we had in terms of application development and modeling were an outcome from such collaborations. Pooling our expertise and know-how would definitely help us get there much sooner than if we tread the same path individually.”
Pitenis concludes that tribology alone offers only a glimpse of the full picture and agrees with Pondicherry on the importance of collaboration. “Exactly how biological systems respond to tribological testing might, in some cases, be more important and more challenging to understand,” she says. “Bridging the tribology and biology communities is key to tackling fundamental questions in biotribology and developing biocompatible material systems for next-generation medical devices, implants, drug delivery systems and therapeutics.”
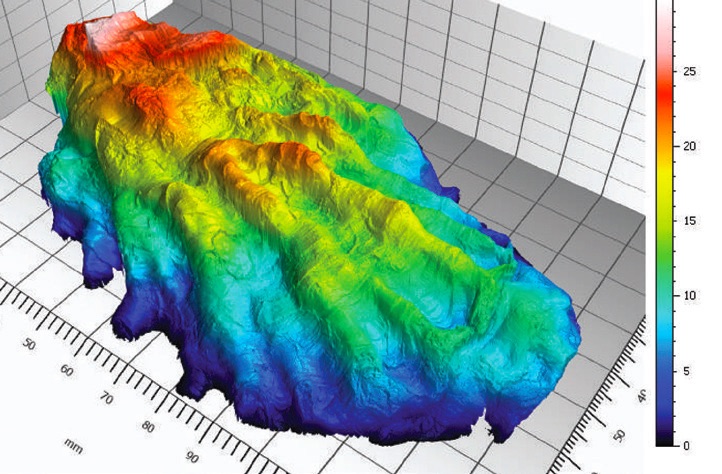
Figure 3. Oyster shell scan, obtained by Nanovea Labs using Nanovea Optical Profilers. Figure courtesy of Nanovea.
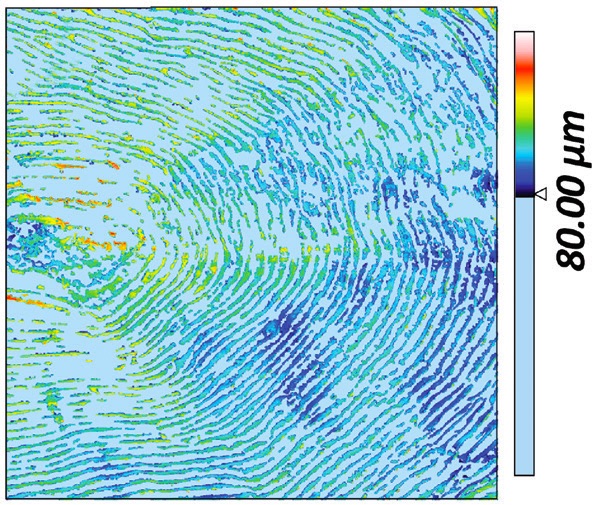
Figure 4. Fish scale scan, obtained by Nanovea Labs using Nanovea Optical Profilers. Figure courtesy of Nanovea.
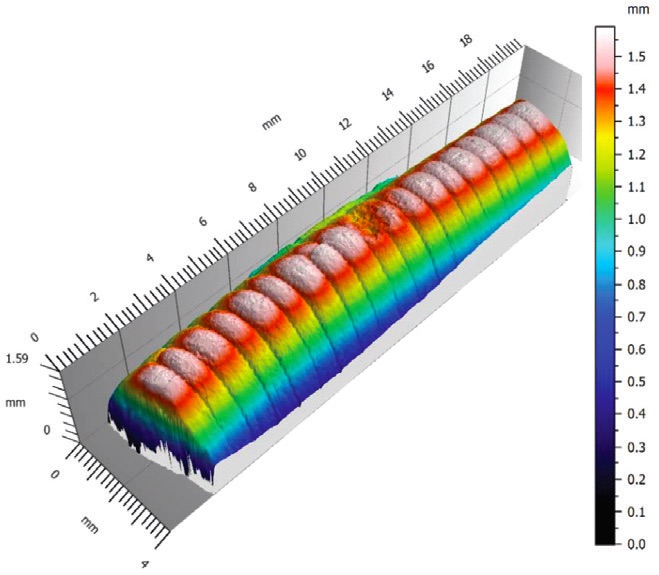
Figure 5. Crinoid stem fossil scan, obtained by Nanovea Labs using Nanovea Optical Profilers. Figure courtesy of Nanovea.
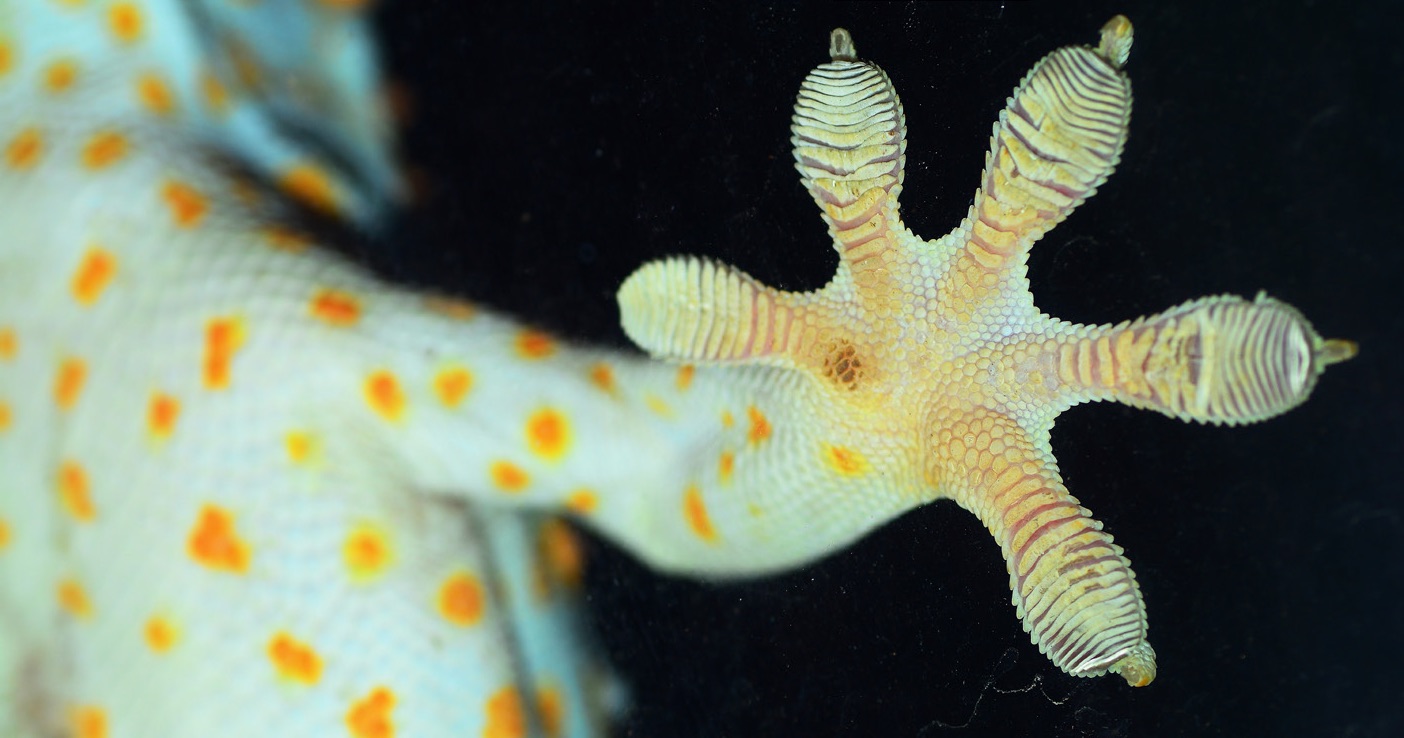
The biotribology of animals6
Three primary types of animal surface interactions that have been studied are fish/water, birds/air and burrowing animals/soil.
Some animals, such as tree frogs, geckos and insects, can ambulate on inverted vertical surfaces. Much biotribology research has been conducted into understanding the adhesion mechanism of attachment and detachment regarding these animals.
Some say the attachment mechanism is due to capillary force (the ability to conform to a narrow space) while others think van der Waals forces7 are responsible for gecko adhesion in particular—since tiny structures on the bottom of the feet allow it to make very close contact with the surface. When a gecko toe detaches, the high adhesion friction reduces rapidly to a very low value as the gecko rolls its toes upward and backward. Both the adhesion and friction forces can be rapidly changed to accommodate swift attachment and detachment.
A water strider’s legs are extremely hydrophobic. This allows it to float and even run on water. Biotribology research involving animals, such as the gecko and water strider, contributes to bionic design theory and the manufacture of products that mimic these properties.
Much work has been undertaken to fabricate synthetic surface structures that mimic unique animal surface functions.8
REFERENCES
1. Haptics is the study of the sense of touch to perceive and manipulate objects and the sense of movement and position of the body.
2. Dowson, D. (1969), “Review Paper 2: Whither Tribology?” Proc. Inst. Mech. Eng. Conf. Proc. 184, pp. 181-185. Doi:10.1243/PIME_CONF_1969_184_384_02. Available here.
3. Abubacker, S., McPeak, A., Dorosz, S.G., Egberts, P. and Schmidt, T.A. (2018), “Effect of counterface on cartilage boundary lubricating ability by proteoglycan 4 and hyaluronan: Cartilage-glass versus cartilage-cartilage,” J Orthop Res. Available here.
4. Available here.
5. Schmidt’s paper available here.
6. From “Biotribology: Recent progresses and future perspectives.” Available here.
7. Van der Waals forces are the attractive forces that hold molecules in close proximity to each other, regardless of whether they are polar or non-polar. Their charge fluctuations naturally fall into sync, spontaneously creating an attractive field.
8. Additional information available here and here.
Jeanna Van Rensselar heads her own communication/public relations firm, Smart PR Communications, in Naperville, Ill. You can reach her at jeanna@smartprcommunications.com.