Sodium-ion batteries are under evaluation as potential alternatives to lithium-ion batteries.
The cycle life of sodium-ion batteries has improved through the use of a new electrolyte known as sodium bis(fluorosulfonyl)imide and a new nickel-rich O3-layered transition metal oxide also based on manganese and cobalt.
Battery performance, as evaluated by the specific cathode capacity, increased in part due to suppression of the build-up of inactive sodium crystals on the surface of this electrode.
The movement to develop electric vehicles powered by batteries is ongoing. Lithium-ion batteries have emerged as the leading choice at this time.
As previously discussed in Tech Beat, lithium-ion batteries have demonstrated performance limitations. One of the key concerns is safety issues focused on the current use of a non-aqueous, organic solvent that has been found to be the cause for flammability, moisture sensitivity and toxicity.
In a previous TLT article,
1 researchers developed an aqueous lithium-ion battery based on an aqueous water-in-salt electrolyte and a new niobium tungsten oxide anode that replaces graphite. The researchers found that this approach led to the best volumetric performance observed in an aqueous lithium-ion battery to date. Using water in the electrolyte reduces the safety concerns, but battery performance still needs to be optimized for this idea to be considered for commercialization.
Sodium-ion batteries have been considered to be potentially viable for use in applications such as electric vehicles. Dr. Junhua Song, a graduate of Washington State University in Pullman, Wash., says, “Sodium-ion batteries should be under consideration for use because they are prepared from raw materials that are cheaper and more readily available than lithium-ion batteries. For examples, sodium is a relatively abundant and low-cost raw material. In contrast, lithium and cobalt (the primary component in lithium-ion battery cathodes) are more expensive and not as readily available.”
One other benefit for sodium-ion batteries is compatibility with aluminum. Song explains, “Aluminum is the favorite choice for use as current collectors in batteries due to its low cost and light weight. The problem in lithium-ion batteries is that aluminum can form alloys with lithium, leading to corrosion, which can negatively impact performance. Sodium does not alloy with aluminum, meaning that the currently favored current collector can be used without any incompatibility concerns.”
Sodium-ion batteries do not exhibit the energy density of lithium-ion batteries and cannot be as effectively recharged. These factors have hindered their development. A second problem that sodium-ion batteries face is finding a suitable layered cathodic material. During operation, a layer of inactive sodium crystals builds up on the surface of the cathode, which restricts the flow of sodium ions and, in effect, prevents the battery from operating.
A cathodic material that shows promise in sodium-ion batteries is O3-layered metal oxides based on transition metals. Song says, “These materials exhibit properties that are similar to the cathode used in lithium-ion batteries. The O3 designation refers to the crystallographic location of sodium ion and stacking order of the transition metal layers.”
Those O3-layered transition metal oxides that have been examined have not provided good performance due to irreversible phase transition at high voltages and poor cycle life. A new electrolyte has now been developed that improves the cyclability of layered metal oxide cathodic materials in sodium-ion batteries.
Song, Yuehe Lin, professor in Washington State University’s School of Mechanical Engineering and Materials Engineering, and Xiaolin Li and his colleagues at Pacific National Laboratory synthesized a nickel-rich O3-layered transition metal oxide (also based on manganese and cobalt), that shows promise as a cathode in a sodium-ion battery. Song says, “We targeted a nickel-rich oxide because this has the potential for more battery capacity. The relatively high nickel containing cathode produced a layered structure with attractive energy density.”
The researchers tried to make the structure of the cathodic material similar to a lithium-ion battery. Song says, “We realized that sodium ions are larger than lithium ions, making it difficult for them to squeeze between layered structures and cause irreversible phase transition. We developed a new electrolyte formula to control the surface layer transition between the cathode and the electrolyte, which is known as the cathode electrolyte interphase.”
The nickel-rich O3-layered transition metal oxide was prepared by a co-precipitation method, according to Song. He says, “We used sodium hydroxide and sodium carbonate to infuse sodium ions into the layered structure. The primary metal oxide particles ranged in size from 100 to 200 nanometers.”
The researchers evaluated the cathode in a sodium-ion battery that used a standard, hard carbon anode. A specific cathode capacity of 196 milliampere hours per gram and a greater than 80% retention of performance over 1,000 cycles was achieved. This result represents a step forward in the potential commercialization of sodium-ion batteries.
A 60 milliampere hours pouch cell was prepared and displayed stable performance. The cell is shown in Figure 2.
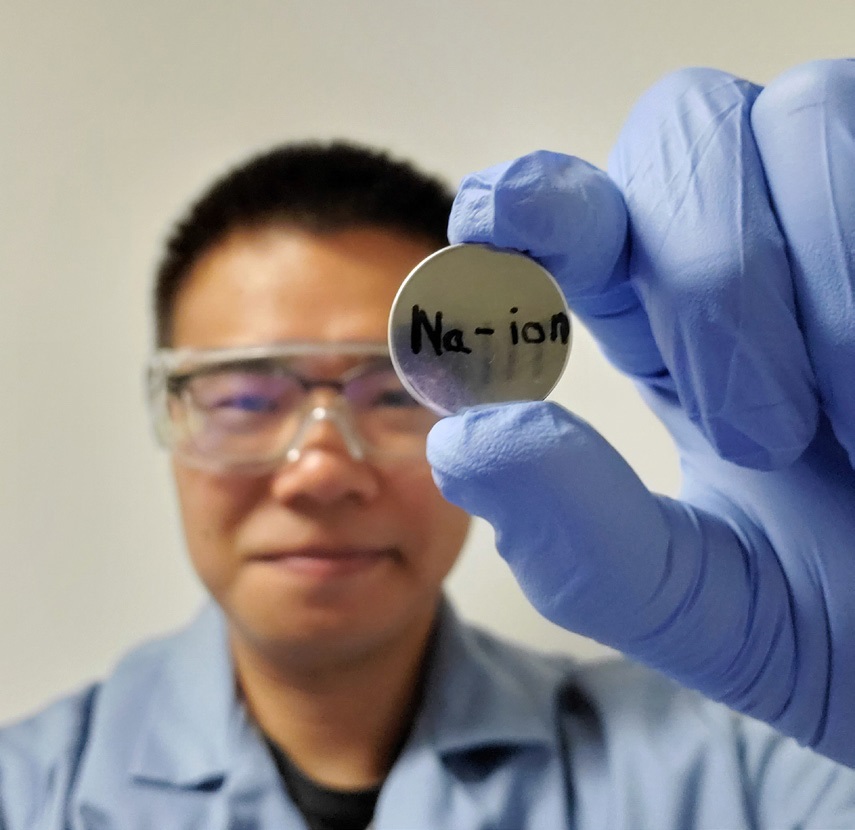
Figure 2. A sodium-ion battery prepared with a new electrolyte and new cathodic material was evaluated in a 60 milliampere hours pouch cell and found to exhibit stable performance. Figure courtesy of Washington State University.
The success of the sodium-ion battery was due in part to suppression of the build-up of inactive sodium crystals on the surface of the cathode. An advanced electrolyte based on sodium bis(fluorosulfonyl) imide played an important role in the performance of the battery. Song says, “This electrolyte exhibited a solvating power that led to a higher concentration of salt. This changed the solvation structure facilitating higher performance.”
The researchers will be working to improve the efficiency of the sodium-ion battery in the future. Song says, “We will be switching from a 200 milliampere hours per gram hard anode to a 400 milliampere hours per gram hard carbon anode. Other strategies include optimizing the electrolyte and eliminating the use of cobalt in the cathodic material.”
Additional information can be found in a recent article
2 or by contacting Lin at
yuehe.lin@wsu.edu or Li at
xiaolin.li@pnnl.gov.
REFERENCES
1. Canter, N. (2020), “Aqueous lithium-ion battery,” TLT,
76 (3), pp. 12-13.
2. Song, J., Wang, K., Zheng, J., Engelhard, M., Xiao, B., Hu, E., Zhu, Z., Wang, C., Sui, M., Lin, Y., Reed, D., Sprenkle, V. and Yan, P. (2020), “Controlling Surface Phase Transition and Chemical Reactivity of O3-Layered Metal Oxide Cathodes for High-Performance Na-Ion Batteries,”
ACS Energy Letters, 5 (6), pp. 1718-1725.