Stretchable batteries for wearable electronics
Dr. Neil Canter, Contributing Editor | TLT Tech Beat April 2020
A monomer based on 2-ureido-4 pymidone was incorporated into a supramolecular lithium-ion conductor that can be used in stretchable batteries.
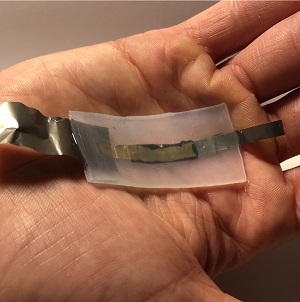
Figure 2. An image of a supramolecular lithium-ion conductor is shown that can be used in stretchable batteries. Figure courtesy of Stanford University.
KEY CONCEPTS
- A supramolecular lithium-ion conductor (SLIC) has been developed that exhibits both strong ionic conductivity and mechanical properties.
- Reversible hydrogen bonding in the SLIC is responsible for the ability of the material to deform without breaking and for enabling a smooth interface between the electrode and electrolyte.
- Addition of a polymer electrolyte, an anode and a cathode to the SLIC produced a stretchable battery with 70% active material.
Lithium-ion batteries are under development and evaluation for a number of applications. This column has been paying close attention to how lithium-ion batteries are being designed for use to power electric vehicles. But other applications for lithium-ion batteries are under development including for wearable electronics such as watches that are in close proximity to the human body.
In a previous TLT article (1), researchers found that stretchable batteries could be developed through a process known as screen printing. An ink based on zinc-silver oxide was mixed with a polystyrene-polyisoprene-polystyrene (SIS) block copolymer and then screen printed on a stretchable textile. No lose in performance was found after the screen-printed battery was stretched to an elongation of 100%.
The researchers wanted to work with lithium batteries but were concerned about their flammability. This issue has led researchers to evaluate the use of solid electrolyte materials as alternatives to flammable, liquid electrolytes.
David Mackanic, graduate student at the University of Stanford in Palo Alto, Calif., says, “Preparation of a solid electrolyte requires designing a material that is very ionically conductive and has strong mechanical properties. One option has been to implement ceramic materials, but, unfortunately, they are brittle and difficult to work with despite their high ionic conductivity.”
Mackanic has another consideration in working with any material. He says, “A candidate material must have a smooth and well-integrated interface from cathode to electrolyte to anode.”
Polymeric electrolytes have been under evaluation for this application, but one of the challenges has been finding a resin that does not lose mechanical properties in order to increase ionic conductivity. The problem faced in evaluating most polymers is that reducing the glass transition temperature increases ionic conductivity but leads to a reduction in mechanical properties.
Mackanic says, “The leading polymeric electrolyte is a polystyrene-polyethylene oxide block copolymer. Mechanical properties are contributed by the polystyrene, while the polyethylene oxide imparts ionic conductivity.”
The problem for this copolymer and all other materials is a lack of elastic flexibility, making them unsuitable for use in wearable electronics which require stretchability. A new material has now been developed that not only resolves the flexibility issue but also exhibits strong ionic conductivity.
Hydrogen Bonding
Mackanic and his colleagues have developed a material known as a supramolecular lithium-ion conductor (SLIC) (see Figure 2) that exhibits both strong ionic conductivity and the mechanical properties that operate independently of each other. Ionic conductivity is contributed by the low glass transition temperature derived from a poly (propylene glycol)-poly (ethylene glycol)-poly (propylene glycol) block copolymer. Mechanical strength was contributed by copolymerizing with a monomer based on 2-ureido-4-pyrmidone (UPy).
Mackanic says, “The key to the performance of the SLIC is the hydrogen bonding present in the UPy group. This hydrogen bonding is used in a similar fashion in the double helix structure that is characteristic of DNA. Hydrogen bonding enables the UPy groups to exhibit strong mechanical properties including excellent toughness and elasticity. Most importantly, the hydrogen bonds are reversible, enabling the SLIC to undergo large amounts of deformation without breaking. These dynamic hydrogen bonds also allow for uniform interfaces between the electrode and electrolyte.”
The researchers determined the influence of the UPy group by preparing the SLIC with 0% hydrogen bonding units (SLIC-0) and SLIC-3 with 100% hydrogen bonding units. The intermediate structures, SLIC-1 and SLIC-2, contained some hydrogen bonding units as well as aliphatic carbon chain structures.
Mackanic says, “In progressing from SLIC-0 to SLIC-3, hydrogen bonding was slowly added to the polymer backbone. SLIC-0 acted as a traditional polymer with inferior mechanical properties. As more hydrogen bonding was added, the SLIC’s elastic properties steadily improved along with the polymer’s toughness.”
To evaluate the effectiveness of the SLIC, polymer electrolytes were produced by dissolving the lithium salt, LiTFSI (lithium bis (trifluoromethanesulfonyl) imide) into the polymer and casting a film. The LiTFSI caused a slight decrease in the mechanical properties of the polymer necessitating the use of additives.
Mackanic says, “The large size of LiTFSI’s anions was found to interfere with the SLIC’s chain packing, reducing the effectiveness of the hydrogen bonding. To counteract this reduction in performance, silicon dioxide was added to improve not only the mechanical properties but also the ion transport properties.”
The unique properties of the SLIC enabled the researchers to use it as a stretchable electrode binder material. Mackanic says, “SLIC, lithium iron phosphate (the cathode) and lithium titanate (anode) were mixed into a slurry to produce a stretchable battery with free-standing electrodes.
A stretchable battery with a capacity of 1.1 milliampere hours per square centimeter was prepared that contained electrodes with 70% active material. This battery was functional when stretched up to 70% strain.
Mackanic says, “Future work will involve preparing the stretchable battery with no additional additives, improving energy density and evaluating different active materials. We are also exploring commercial applications.”
Additional information can be found in a recent article (2) or by contacting ackanic@stanford.edu.
REFERENCES
1. Canter, N. (2017), “Printing batteries,” TLT, 73 (8), pp. 10-11.
2. Mackanic, D., Yan, X., Zhang, Q., Matsuhisa, N., Yu, Z., Jiang, Y., Manika, T., Lopez, J., Yan, H., Liu, K., Chen, X., Cui, Y. and Bao, Z. (2019), “Decoupling of mechanical properties and ionic conductivity in supramolecular lithium ion conductors,” Nature Communications, 10, Article Number: 5384.
Neil Canter heads his own consulting company, Chemical Solutions, in Willow Grove, Pa. Ideas for Tech Beat can be submitted to him at neilcanter@comcast.net.