20 Minutes with Daniela Fritter
Rachel Fowler, Managing Editor | TLT 20 Minutes March 2020
This physical chemist with Clorox uses tribology to solve problems with personal care, food and cleaning products.
Daniela Fritter - The Quick File
Daniela Fritter is an Associate Research Fellow in the Advanced Measurement Sciences department of Clorox R&D. She earned her doctorate in physical chemistry at the University of California, Los Angeles, studying the evolution of droplet patterns on surfaces through growth and coalescence (breath figures). In post-doc work for the Air and Industrial Hygiene Lab in Berkeley, Calif., she investigated particle resuspension and deagglomeration in a model system to predict the effect of impaction in air samplers.
Fritter began her career at Clorox in 1991 working on upstream technology for hard surface cleaning and laundry and fat replacement in salad dressings. She also spent three years in product development in the Hidden Valley group, leading multifunctional teams in bringing salad dressing line extensions and product improvements to market. Since 2002 she has been the lead rheologist for Clorox, providing technical guidance and insights to enable optimization and innovation in product categories across the portfolio. In that time, she also has built tribology capability in cleaning, foods and personal care applications, owing to the overlap between the two disciplines. Fritter holds patents on surfactant thickening of cleaning gels, delivery of oil from polymer matrices and hand grippability of cleaning pads. She is a member of ACS, AOCS, AIP, SOR, STLE and SWE.
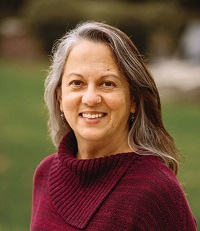
Daniela Fritter
TLT: How did you get interested in rheology and tribology?
Fritter: As a physical chemist, I’ve always been curious about the phenomena that underlie macroscopic observable behavior and appreciative of physical models that both explain what’s going on and provide the practical levers to pull to make desired changes. This mindset works well in the CPG (consumer packaged goods) industry where the consumer’s sensory experience is an important component of overall product performance: how it looks in the bottle, the scent on removing the cap, how it pours, how quickly the stain disappears.
I was first drawn to rheology, the study of how materials deform or flow under stress, because it provided a way to quantitate practical behavior I directly experienced with my own eyes or hands—appearance and feel of the product on shaking or dispensing or rubbing it on surfaces, cling to vertical surfaces, ease of spraying, etc. But rheology also has a theoretical underpinning that addresses how colloidal forces interact with the ingredients and the mixing process to form a product’s microstructure, providing the link between the molecular and the macroscopic that allows us to design and optimize products for manufacturability and consumer preferred flow.
While much of the consumer’s real-time interaction with a product is rheological in nature, it’s sometimes necessary to invoke tribology to explain some new and unexpected behavior when the moving surfaces come close enough to interact. A personal care product might pull more on the skin during application, or a salad dressing might have a fattier mouthfeel, in a way that is not predicted by rheology alone. Cleaning wipes made with the same substrate and cleaning fluid can be experienced very differently as they’re pulled across different surfaces or soils. Such phenomena often have tribological roots.
TLT: What are the methodologies you use to measure performance?
Fritter: We have technical performance criteria and associated manufacturing specifications across all of our product forms to ensure consistency in product quality and consumer satisfaction. These might concern things like cleaning performance, antimicrobial efficacy, packaging stability, product shelf life and aesthetic features like color, fragrance and viscosity. We generally align with CPG industry standards but also develop in-house proprietary methods to meet specific analytical and predictive needs. Because the CPG industry is experiential and sensory driven, many of our challenges involve translating qualitative product attributes into hard numbers that relate to the consumer experience in a meaningful way.
With respect to rheology, an empirical spindle-in-beaker viscometer is commonly used to assess simple formulation or process changes, track stability or for manufacturing quality control. However, this type of measurement typically does not account for shear rate dependence and might not capture important consequences of more complicated changes to the product matrix. For this purpose, a stress-controlled rheometer with a concentric cylinder and either cone-and-plate or parallel plate geometries is a standard tool for more in-depth flow characterization of thickened fluids and soft solids.
In viscometry mode, a material is sheared in rotation until a steady-state viscosity is reached. A profile of the viscosity as a function of shear rate describes the general flow behavior of the material under different practical conditions. Low shear rates correspond to flow under gravity or suspension ability; medium shear rates correspond to shaking, stirring and pouring; high shear rates correspond to pumping, spraying and hydrodynamic lubrication (involving always a fully intact fluid film between the surfaces). In dynamic mode, small oscillations are applied to map the viscoelastic material response, allowing deeper insights into interactions between particles and microstructural behavior. Characterizing parameters such as relaxation time or critical strain can be related to actives delivery or gel-like appearance.
Rheological measurement is based on well-defined boundary conditions and long-established equations of fluid flow; scaling relationships and prediction of practical behaviors are reasonably well understood for many types of systems. With tribology, physical characterization is more complicated and chemistry specific, often involving multiple phenomena, multiple scales and temporal changes including wear during the course of measurement. This results in a variety of empirical tools and techniques that are highly specific to the problem being studied. In the realm of consumer products, for example, widely different methods have been used for assessing the effect of floor cleaners on slip resistance,1 the effect of shampoos and conditioners on the feel of human hair,2 sensory perception of food products in the mouth3 and personal care products on the skin4 and mechanisms of hard surface cleaning.5
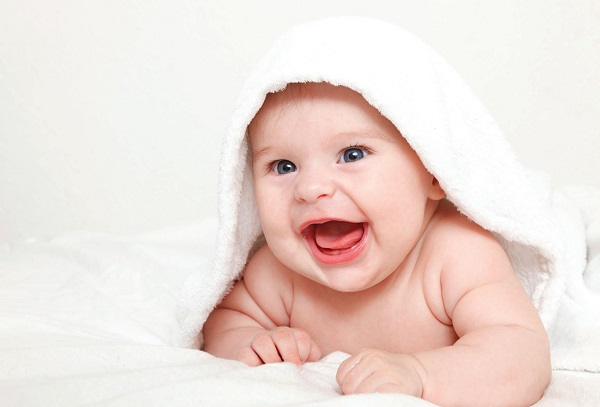
There is still much we don’t understand about the tribological behavior of human skin.
TLT: Describe a tool or method you used to solve a tribological problem.
Fritter: One of our technical teams was developing cleaning pads for use on kitchen or bathroom surfaces. The pad is dry to the touch but impregnated with cleaning ingredients that release when wetted with water. The resulting suds can migrate between the hand and the pad, causing the hand to slip during cleaning. The team noticed that incorporating natural pulp fiber into the hand-facing layer resulted in better grip during cleaning, and wanted to validate this benefit with technical data rather than spend money on consumer testing. So I developed a method to quantitate the hand gripability of the cleaning pad in a reproducible and statistically discriminating way, while emulating as closely as possible real-use conditions by using human operators and a platform on load cells that can accommodate a human hand in a wiping motion.
The cleaning pad was primed in a controlled way to wet it and release the suds, then affixed to the platform with the hand-facing side up. Applying some pressure, the operator slid his or her hand back and forth across the immobilized pad to generate lateral and vertical forces from which sliding friction coefficients were calculated. To minimize variability and focus the work, our protocol specified a narrow range of vertical force and speed within a consumer relevant window. The operator monitored the applied vertical force and made adjustments in real time to stay within a 1-5-pound range. The path length of the reciprocating motion was fixed, as was the timing of each back-and-forth stroke, resulting in a velocity between 15-20 cm per second.
The values of friction coefficient collected in this way were reproducible by the same operator. In addition, the same relative product differences were seen by all operators, although the values for Operator 3 were systematically higher (see Figure 1a). We can attribute this to the larger hand size of Operator 3 resulting in a lower contact pressure by virtue of keeping the applied vertical force constant across operators. A lower contact pressure translates for wet skin into a higher friction coefficient.6 This suggests that changing the method to standardize the contact pressure instead of the applied vertical force could remove the bias due to hand size.
Alternatively, we accounted for the hand size difference by applying a scaling factor to the friction coefficient, thus obtaining statistical differentiation to quantitatively demonstrate the effect of pulp. Figure 1b shows the scaled variable of Hand Grip across three operators for a series of cleaning pads in which the hand-facing layer ranged in pulp content from 0%-70%. This and other data allowed us to make a technical claim for proprietary advantage,7 specifically that the use of natural pulp at levels of at least 40% in the hand-facing layer lessens slip of the cleaning pad in the hand, ultimately translating into a better consumer experience.
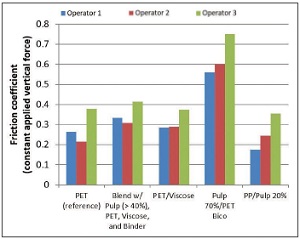
Figure 1a. Sliding friction coefficients obtained with human operators sliding their hands across wetted and immobilized cleaning pads under controlled conditions (applied vertical force between 1 and 5 pounds, reciprocating path length 3.5”, 1 second per back-and-forth cycle). Cleaning pads are of similar composition and construction except for the hand-facing layer, which contains the test compositions of synthetic-pulp blends as labelled in the chart.
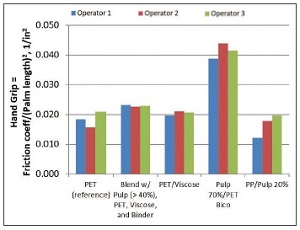
Figure 1b. The data of Figure 1a, where the friction coefficient has been divided by the square of the palm length of each operator, creating a new variable called hand grip. (Palm length is 3.80” for Operator 1, 3.70” for Operator 2 and 4.25” for Operator 3.) The two materials with at least 40% pulp have statistically higher hand grip versus PET and PET/viscose (synthetic/semisynthetic materials containing 0% natural pulp). Hand grip is significantly higher for the blend with >40% pulp versus either PET or PET/viscose, using t-test of values across evaluators at 90% confidence.
TLT: What area would you most like to explore?
Fritter: There is much we still don’t understand about the tribological behavior of human skin, a soft biomaterial characterized by nonlinear viscoelastic material response and a complex surface structure. The main contributor to skin frictional behavior is thought to be adhesion friction caused by attractive surface forces at the skin-material interface, while deformation usually plays a lesser role. I’d like to better understand the nature of the localized adhesion forces during skin contact with a given surface, and which chemical and geometric factors have the biggest influence. I see this as analogous to understanding how colloidal forces influence aggregation to link the molecular to the macroscopic in rheological flow.
Testing with human subjects (in-vivo) still provides the most realistic results, but ultimately being able to move to more controlled and sophisticated in-vitro measurements would save time and increase the scope of variables that can be investigated, and with higher precision. This could be achieved with better polymeric skin mimics and sensory feedback loops that more closely approach the human response, coupled with robotics and AI to handle the larger data processing requirements.
You can reach Daniela Fritter at daniela.fritter@clorox.com.
REFERENCES
1. ANSI A137.1 Tile Slip Test.
2. Whitby, R.D. (2007), “Tribology and hair care,” TLT, 63 (3), p. 64.
3. Kun Liu PhD thesis (2016), “Lubrication and perception of foods – Tribological, rheological and sensory properties of particle-filled food systems,” Wageningen University.
4. Horiuchi et al. (2009), “Relationship between tactile sensation and friction signals in cosmetic foundation,” Tribology Letters, 36 (2), pp. 113-123.
5. Luetkenhaus et al. (2016), “The Tribology of Cleaning Processes,” Tribology Online, 11 (2), p. 298.
6. Derler, S. and Gerhardt, L. (2012), “Tribology of Skin: Review and Analysis of Experimental Results for the Friction Coefficient of Human Skin,” Tribology Letters, 45, pp. 1-27.
7. US Patent 10,219,672, Multilayer Cleaning Article With Gripping Layer and Dry Surface Contact Layer, Elisa Calimano, Daniela Fritter, Kristina Perlas, Jason White, Chosu Khin, Janiece Hope, Bernard Hill, Stephen Fisher, Kelly Dickson, William Ouellette. Available here.