What’s so bad about foamy foams?
Dr. Robert M. Gresham, Contributing Editor | TLT Lubrication Fundamentals November 2018
Plenty. Fortunately, formulators have several bubble-bursting options.
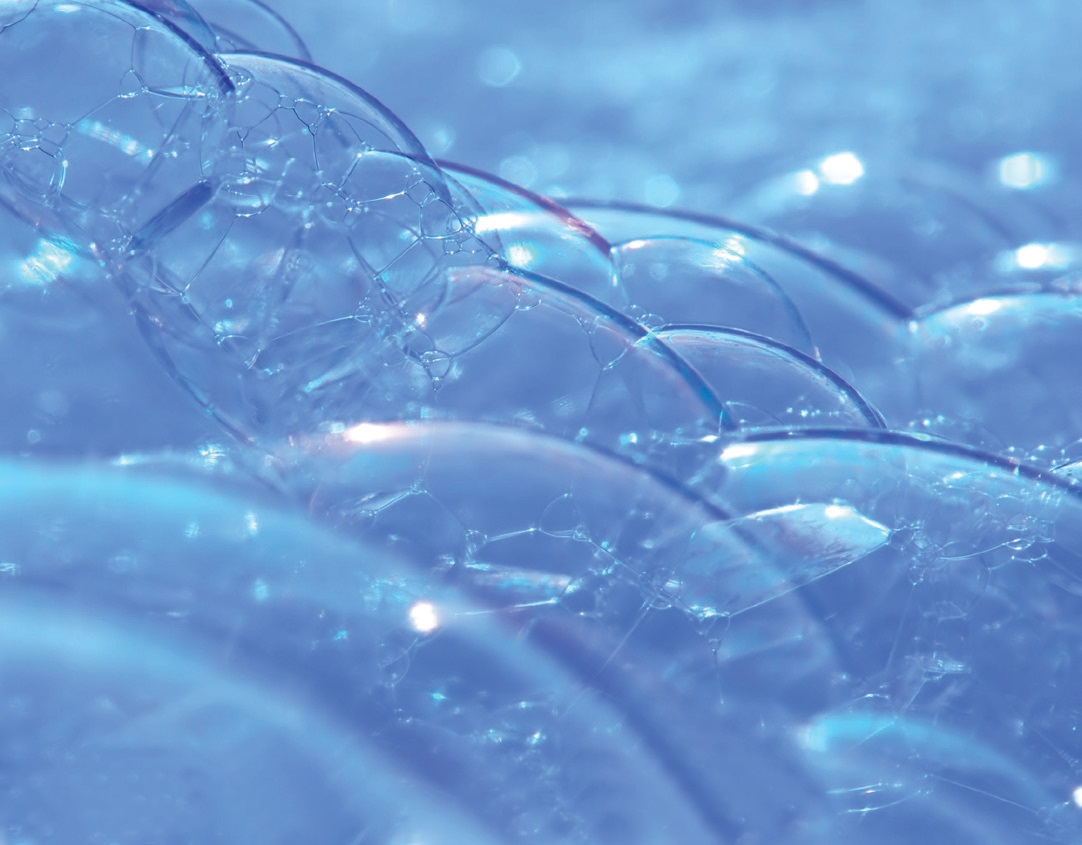
© Can Stock Photo / Linnea
Surfactants are a fascinating class of chemistries. Their uses—whether in nature or through Man’s interaction—can seemingly do the impossible, such as cause oil and water to “mix,” form stable emulsions like milk, the list is endless.
In this column, I’ve often talked about surfactants and their importance as dispersants and detergents in lubricants; and how, in low concentrations, surfactants can act as an antifoam; or, in greater concentrations, as a wetting agent (to eliminate floating swarf in metalworking operations); or in yet higher concentrations make a stabile foam (such as shaving cream).
Foam is essentially a dispersed gas in a liquid. In lubricants and metalworking fluids (MWFs), uncontrolled foam, ambient air entrained or dispersed in the lubricant, can have very adverse effects. For both aqueous and non-aqueous MWFs and lubricants, foam can cause the fluid film to break down, impair heat removal and increase the rate of oxidation of the fluid, cause cavitation in pumps resulting in poor fluid flow, pressure variation in hydraulic systems and even loss of fluid through vents—all bad.
While perhaps a fine distinction, we refer to antifoams as materials added to a formulation to prevent or suppress foam as it forms. Defoamers are materials added during the use of fluids to break up foam and to, at least for some time period, suppress foam formation until more needs to be added. In reality, defoamers and antifoams are very similar chemistries and perform similar functions.
Looking at non-aqueous lubricants like gear oils, transmission fluids, etc., we see that foam is generally not thermodynamically stable; that is, given enough time the dispersed or entrained air will separate from the fluid. The issue is the speed of this process versus the process of creating foam, usually through mechanical action. A foam bubble has two interfaces where the outside of the bubble contacts the fluid and where the bubble contacts another bubble or the atmosphere. These interfaces, along with the fluid itself, are referred to as lamella. For a foam bubble to break, the fluid in the lamella must drain sufficiently so the pressure inside the bubble exceeds the strength of the lamella fluid film to hold together. Thus, control of foam is related to inducing conditions that encourage and speed this process.
At the risk of oversimplification, imagine a single foam bubble sitting on top of a fluid. The bubble is defined by a thin layer of the fluid surrounding the air in the bubble. If there is some mechanism that causes this thin fluid layer to become thinner to the point that the air pressure in the bubble exceeds the strength of the fluid to remain a cohesive film surrounding the air in the bubble, the bubble breaks. So what are the factors that affect this process?
Still imagining our foam bubble, the idea with an antifoam is that a tiny droplet of antifoam enters this thin layer of fluid that forms the bubble, then spreads to the point of bridging the thickness of the fluid which, in turn, allows for rapid rupture of the fluid layer and, thus, the bubble. This process then has three distinct stages: entering (E), spreading (S) and bridging (B). These three stages, E, S and B, are all dependent on the respective surface tensions of the liquid film, the antifoam and the surface tension of the two. (Remember surface tension is the force that causes water to bead on a freshly waxed car.) In the case of our lubricant foam bubble, the surface tension of the antifoam must be lower than the surface tension of the lubricant fluid surrounding the bubble. Then, where bridging occurs, the bubble no longer has the strength to hold together and has to rupture.
As said, this is an oversimplification; other factors that affect this fundamental process are relatively low solubility of the antifoam in the lubricant fluid and the number and size of the antifoam droplets. Because this adds a considerable layer of complexity to the control of foam, we find that the chemistry, lubricant additives and end-use conditions for a specific system force the choice of antifoam and its consequent droplet size, to be determined by both experience and real-world testing. Other factors that influence this process are the lubricant viscosity and the size of the bubbles themselves, which affect their rise to the surface of the lubricant. Thus, foam in higher viscosity fluids with very small bubbles due to aggressive mechanical action in the system can result in more stable foams that are more difficult to control.
In practice, it is not surprising to find many antifoams available nor surprising that antifoams used in transmissions, gear oils and other mechanical applications will all use very different antifoam products. Further, the design of mechanical systems can exacerbate foam control. Indeed, the method for evaluating antifoam performance must be somewhat tailored to the specific lubricant and its application. Thus, foamy foams are complicated systems and bad for our lubricant systems. Fortunately the formulator has many options to help control this problem.
Bob Gresham is STLE’s director of professional development. You can reach him at rgresham@stle.org.