Grease manufacturing: Chemistry and processes
Dr. Nancy McGuire, Contributing Editor | TLT Webinars March 2017
The right materials and processes can make the difference between success and failure.
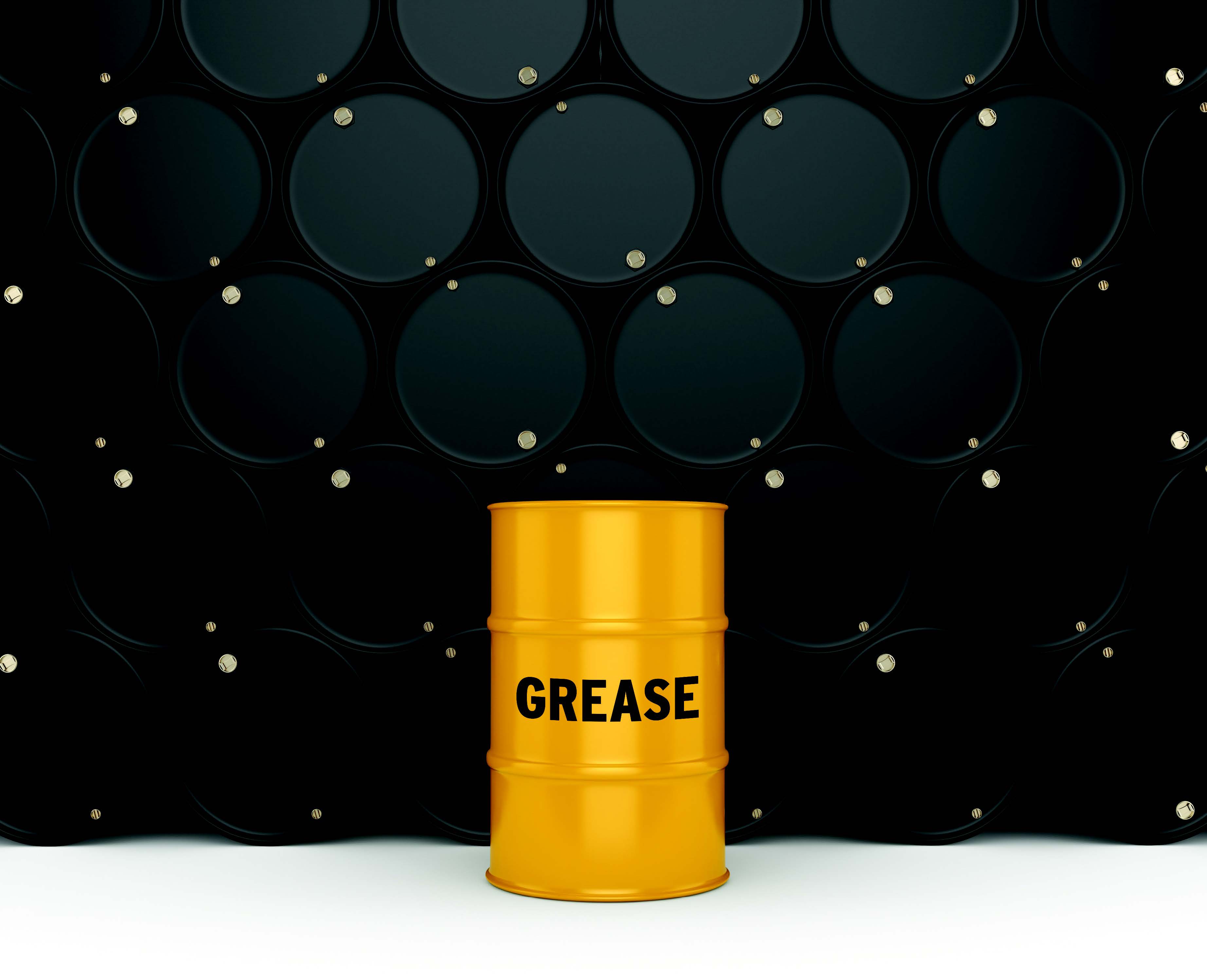
© Can Stock Photo / apopium
KEY CONCEPTS
•
The grease manufacturing process is as important as the formulation in providing the desired properties and performance.
•
Open kettles, pressure kettles, Contactor® vessels and continuous process units each offer advantages for various formulations and processes.
•
Maintaining control of the starting materials and the reaction and processing conditions increases product consistency and process predictability.
MEET THE PRESENTER
This article is based on a Webinar presented by STLE Education on Sept. 27, 2016. “Grease Manufacturing” is available at
www.stle.org: $39 to STLE members, $59 for all others.
David Turner is a product specialist with the Lubricants Fluid Technology Group of CITGO Petroleum Corp. in Houston. He is a graduate of Lamar University in Beaumont, Texas, with a bachelor’s of science in chemical engineering. Turner has more than 35 years of experience in the lubricants industry in the areas of formulation, manufacturing, selection, application and technical service of lubricants and greases. He is an NLGI Certified Lubricating Grease Specialist (CLGS), STLE Certified Oil Monitoring Analyst I™ (OMA I) and an STLE Certified Lubrication Specialist™ (CLS). A member of STLE and ASTM, Turner can be reached at
dturne3@citgo.com.
David Turner
GREASE MANUFACTURING GOES BEYOND BLENDING OILS. The manufacturing process is as important as the formulation in providing the desired properties and performance. A wide variety of applications requires an equally diverse range of grease compositions and physical properties. Grease is typically about 80%-95% base oil, 2%-20% thickener and 0%-15% additives. However, some greases contain as much as 98% base oil and others contain more than 20% thickener.
THICKENER TYPES
As of 2015 more than 90% of greases used metallic soaps as thickeners, and this figure remains relatively constant from year to year (
1). Simple soap greases are based on lithium, calcium or a mixture of lithium and calcium or sodium (or less commonly, barium or aluminum). Complex greases use soaps based on lithium, calcium, sodium, barium or aluminum.
Less common thickeners made from polyurea, clay, silica or polymers may be used in specific applications like food manufacturing or very low-temperature or high-temperature environments. Polyurea, calcium sulfonate and complex thickeners have largely replaced clay thickeners for high-temperature applications.
Simple soaps are most commonly made of a single fatty acid or fatty ester, but some simple soaps use more than one kind. They are relatively easy to manufacture, but the dropping points of the resulting greases are too low for use at high temperatures.
Simple soaps like lithium 12-hydroxystearate are chemically equivalent. No matter who manufactures it, lithium 12-hydroxystearate is always the same chemical compound. Any differences in the performance of greases based on these soaps arise from the manufacturing process (which can affect the material properties of the grease) and various additives that the manufacturer uses.
Complex soaps use one or more fats, plus a short-chain (C7–C10) diacid that acts as a complexing agent. The selection of the complexing agent can produce widely different properties in complex soaps. Manufacturing these soaps takes slightly longer and requires a higher temperature, but the greases made from complex soaps can be used for high-temperature applications like automotive wheel bearings that must withstand the heat produced by disc brakes.
Clay is a naturally occurring mineral product that consists of thin aluminosilicate layers stacked like a deck of playing cards. Clay thickeners are made by first chemically treating the clay to make it oleophilic, then adding a polar activator material to swell the layers and spread them apart, allowing oil molecules to occupy the spaces in between. Thus, making clay-based greases involves dispersing the clay particles into the base oil rather than forming the thickener using a chemical reaction. Bentonite or hectorite clays can be made into food-grade thickeners that can be further certified as kosher or halal.
Reacting an organic diisocyanate with an organic amine forms polyurea, a type of thickener that helps greases last longer at high temperatures. Polyurea contains no metallic elements—only carbon, hydrogen, nitrogen and oxygen—so the resulting greases are more stable against oxidation than metal soap greases. Although the starting reagents are toxic and chemically aggressive, the polyurea end-product is stable and benign enough to use in food-grade greases.
MAKING SOAP THICKENERS
Soaps are made by reacting alkaline chemicals with animal, vegetable or synthetic long-chain (C16–C22) organic acids or esters (e.g., triglycerides). This reaction is called saponification, and it is the same type of chemical reaction that is used to make the sodium soaps used for bath soaps and skin care products.
Lithium or lithium-complex soaps are used as thickeners in about 75% of all greases worldwide (
1). In a typical reaction, lithium hydroxide monohydrate and 12-hydroxystearic acid form lithium 12-hydroxystearate and water.
Water in the reaction mixture comes from several sources, and it must be removed by dehydration before the grease goes into the finishing stage. The alkali is typically added to the mixture in the form of an aqueous solution, a partial solution or a slurry. Lithium hydroxide occurs naturally in its monohydrate form, which releases water when the lithium reacts with the fatty acid. The saponification reaction itself produces water as a reaction product.
OPEN KETTLE PROCESSES
Open kettle manufacturing is probably the simplest, although not the most efficient, way to make grease. Kettles typically have flat unsealed lids with the inside of the kettle at ambient pressure. All of the steps—saponification, dehydration and finishing—often take place in the same kettle (
see Figure 1).
Figure 1. Open kettle manufacturing process. (Figure courtesy of CITGO Petroleum Corp.)
Fatty acids, base oil, alkali metal hydroxides and water are charged into the kettle through a hatch at the top. Partially blended grease is drawn out through a port in the bottom of the kettle, and a pump recirculates it back into the top of the kettle. Once all components are in the grease, the pump sends the grease through a mill, which homogenizes the grease and disperses the soap thickener throughout the mixture. The grease may then go back into the kettle or be pumped to product storage.
Because grease is so viscous and such a good thermal insulator, it does not circulate by convection; it requires vigorous mechanical agitation to mix it. Inside the kettle, mixer blades agitate and blend the mixture, and scrapers help to improve heat transfer at the kettle walls. The mixing blades are attached along the length of a central shaft and they are tilted at 45 degrees in different directions for better mixing action (
see Figure 2).
Figure 2. Agitator blades (horizontal) and scrapers (white vertical rectangles). (Figure courtesy of CITGO Petroleum Corp.)
The kettle itself has a thermal jacket through which steam or heating and cooling oil are circulated to control the temperature inside the vessel. Grease kettles are taller than they are wide, so that heat from the thermal jacket is more easily transferred all the way to the core of the vessel.
After saponification is complete, the grease is dehydrated in preparation for the finishing steps. Additional base oil and additives are mixed in to produce the desired consistency and performance properties. The grease is deaerated to remove air bubbles and it passes through a filter, which removes contaminants and large particles before it is pumped into packaging containers or sent to a storage tank.
Because saponification takes longer under ambient pressure, the open kettle process is not as efficient as other manufacturing processes (
see Table 1). Also, because every step of the process takes place inside the same kettle, the kettle must be cleaned between every batch to prevent additives from a finished batch from contaminating the initial reaction steps of the next batch. Some operations carry out saponification in one kettle and perform the finishing steps in another kettle or kettles. In this case, some carryover between soap batches does not present a problem.
Table 1. Grease Manufacturing Times (hours)
Open kettle processes are good for clay-thickened greases because no chemical reaction is required, just dispersing the clay particles in the base oil. Calcium sulfonate, which is purchased as a finished thickener (or a precursor that requires some degree of reaction), can be mixed with oil in open kettle or pressure kettle processes but not a Contactor® process. Polyurea thickeners are not generally made in open kettle processes.
PRESSURE KETTLE OPERATIONS
Pressure kettle processes take advantage of the faster saponification reaction that occurs at elevated pressure. Pressure kettle lids are sealed domes, which are designed to contain the elevated pressure generated by steam given off during the reaction. Pressure kettles are typically used only for the saponification reaction, and the grease is then pumped to an open kettle for batch completion.
Polyurea greases almost always require a two-kettle process using a sealed reaction vessel and a separate finishing kettle for dilution, additive introduction and homogenization. The amine and isocyanate starting materials are aggressive and toxic and they must be handled carefully. Some grease production plants make polyurea greases in a separate building from their other processes so that they can set up the proper venting and other precautions required for safe operations. Once the polyurea product is made, however, it is very stable and benign. In fact, polyurea greases can be used in food-grade applications.
CONTACTOR®/KETTLE OPERATIONS
A Contactor® reaction vessel is especially designed for saponification, and it can make as many as four batches of soap concentrate in an eight-hour shift (
see Table 1 and Figure 3). This type of reactor is most commonly used to make lithium soap greases, but it also is used for greases thickened with other metal-based soaps, complex soaps or polyurea. Because this is a sealed reactor, less water is needed for the process.
Figure 3. Contactor® and kettle manufacturing process. (Figure courtesy of CITGO Petroleum Corp.)
The Contactor® vessel has a tapered bottom with a high-speed impeller that pulls the reaction mixture down from the center of the vessel, up through the narrow space between inner and outer jackets and back down through the center (
see Figure 4). The contactor vessel is charged with part of the base oil, a small amount of water, the fatty acid and the alkali. Steam from the saponification reaction pressurizes the vessel. After the reaction is completed, the product goes to a finishing kettle where the rest of the base oil and the additives are introduced.
Figure 4. STRATCO® Grease Contactor™ schematic. (Figure courtesy of STRATCO, Inc.)
CONTINUOUS PROCESSES
Continuous process operations offer several advantages over batch processes. The equipment used to make the grease can be smaller for the same or greater product output. Continuous processing offers better control of the operating variables, which results in a more consistent product (
see Table 2). Continuous processes are suitable for a variety of simple soap and complex soap greases, from National Lubricating Grease Institute (NLGI) grade 000 to grade 3 (although grade 2 is optimum).
Table 2. Kettle Versus Continuous Process
Continuous grease units (CGUs) are shop fabricated and skid mounted, so they can be brought to the plant site by truck or ocean transport. CGU operations require less manpower than batch processes—less than one full-time employee per shift—and commercial-sized CGUs can produce 3,000 to 7,000 lbs. of grease per hour.
The process starts when heated liquid fatty acid or ester, alkali solution or slurry and oil are pumped into a multi-pass plug flow reactor (
see Figure 5). The starting materials circulate at high pressure and high temperature and the reaction is completed very quickly.
Figure 5. Continuous grease process. (Figure courtesy of CITGO Petroleum Corp.)
The product then moves to a flash chamber in which elevated temperature and partial vacuum dehydrate the grease. Afterward, additives and additional base oil are pumped into the soap concentrate stream. Finally the mixture moves to a finishing section in which the finished grease is produced. After the grease reaches the desired consistency, it is pumped to product storage. A rework tank captures any off-spec material and gradually works this back through the process to reduce product loss and disposal costs.
GREASE PROCESS VARIABLES
Grease manufacturing is somewhat of an art, but using process controls can make the outcome more predictable. Better control of the starting materials and reaction and processing conditions increases consistency from batch to batch (
see Table 3).
Table 3. Grease Process Variables
The most important factor in grease processing is the temperature versus time profile. For a typical lithium soap open kettle process, the reactants are charged into the vessel at about 38 C (100 F). The temperature is raised to about 82 C (180 F) for the saponification reaction. This is hot enough to drive the reaction and ensure the components mix properly but not so hot that the water evaporates from the mixture. This is important because saponification takes place at the interface between the hydrophobic phase (fatty acid and base oil) and the hydrophilic phase (alkali and water).
When the saponification reaction is complete, the vessel temperature is raised to boil off the water. The temperature stays around 100 C (212 F) until the water has mostly boiled away, and then it rises rapidly to about 204 C (400 F). At this point, the material in the kettle is completely fluid. The temperature is held here for about 15-20 minutes and then the mixture is quenched. Cooler oil is run through the thermal jacket and cooler base oil is added to the soap mixture. The rate of oil addition should be controlled to produce the prescribed cooling rate.
At this stage, the cooling rate is critical because the soap fiber matrix that holds the base oil is beginning to form and grow. When the temperature drops to about 149 C (300 F), rapid cooling is resumed. At this stage, base oil is added as quickly as possible for efficiency reasons but not so quickly that it pools on the top of the grease mass, a situation that should be avoided. Oil is added until the desired consistency is reached and high-temperature additives are introduced.
Additives must be introduced in the right order and at the right temperature. When the mixture reaches about 93 C (200 F), the lower temperature additives are introduced at a rate that allows them to be incorporated into the grease rather than balling up on the surface. For greases with multiple additives, it is important that the concentrated additives not come into direct contact with each other to avoid unwanted interactions. Making sure that one additive is well dispersed into the grease before the next one is introduced can avoid this problem. If an additive cocktail is to be used, the compatibility of the additives in concentrated form and the age stability of the cocktail must be determined. Once the grease cools to about 82 C (180 F), it can be pumped into packaging or into product storage.
Homogenization severity affects the amount of soap or other thickener required to obtain the desired consistency. Over-homogenized grease can have poor mechanical stability, and excessive oil may separate from the soap matrix during operations. Under-homogenization results in too much thickener in the product, which raises costs and may cause poor pumping properties and poor handling at low temperatures.
The best soap thickeners are those where the fatty acids have been completely saponified because the fatty acids themselves are not good thickeners. To drive the saponification reaction to completion requires an excess of the alkali compound, so the reaction is usually conducted under slightly alkaline conditions.
Base oils can be naphthenic or paraffinic mineral oils, or they can be polyalphaolefins, synthetic or natural esters, silicone oils or other synthetic fluids. The nature of the base oil will affect the amount of thickener needed and the ease of producing the grease.
FROM ART TO SCIENCE
Grease end-users rely on these specialized lubricants to keep their operations going under a wide range of temperatures, pressures, chemical and physical environments and regulatory constraints. A grease that works well for automotive wheel bearings isn’t suitable for a food production line, an anchor chain for a ship or the tracks on an overhead crane. Customers also want a specific formulation and grade of grease to perform the same way with every batch. Choosing the right starting materials and process conditions and paying close attention to process variables can make the difference between success and failure in grease manufacturing.
ACKNOWLEDGMENTS
Chuck Coe, CLGS, CLS – Grease Technology Solutions LLC
John Kay, P.E., CLGS – Stratco, Inc.
REFERENCE
1.
2015 NLGI Lubricating Grease Production Survey. Available at
www.nlgi.org/products-page/2015-grease-production-survey.
Nancy McGuire is a free-lance writer based in Silver Spring, Md. You can contact her at nmcguire@wordchemist.com.