How basestocks and additives are improving energy efficiency
Jean Van Rensselar, Contributing Editor | TLT Cover Story October 2011
As more manufacturers are discovering, substantial gains in fuel economy can be realized with the right lubricant and package.
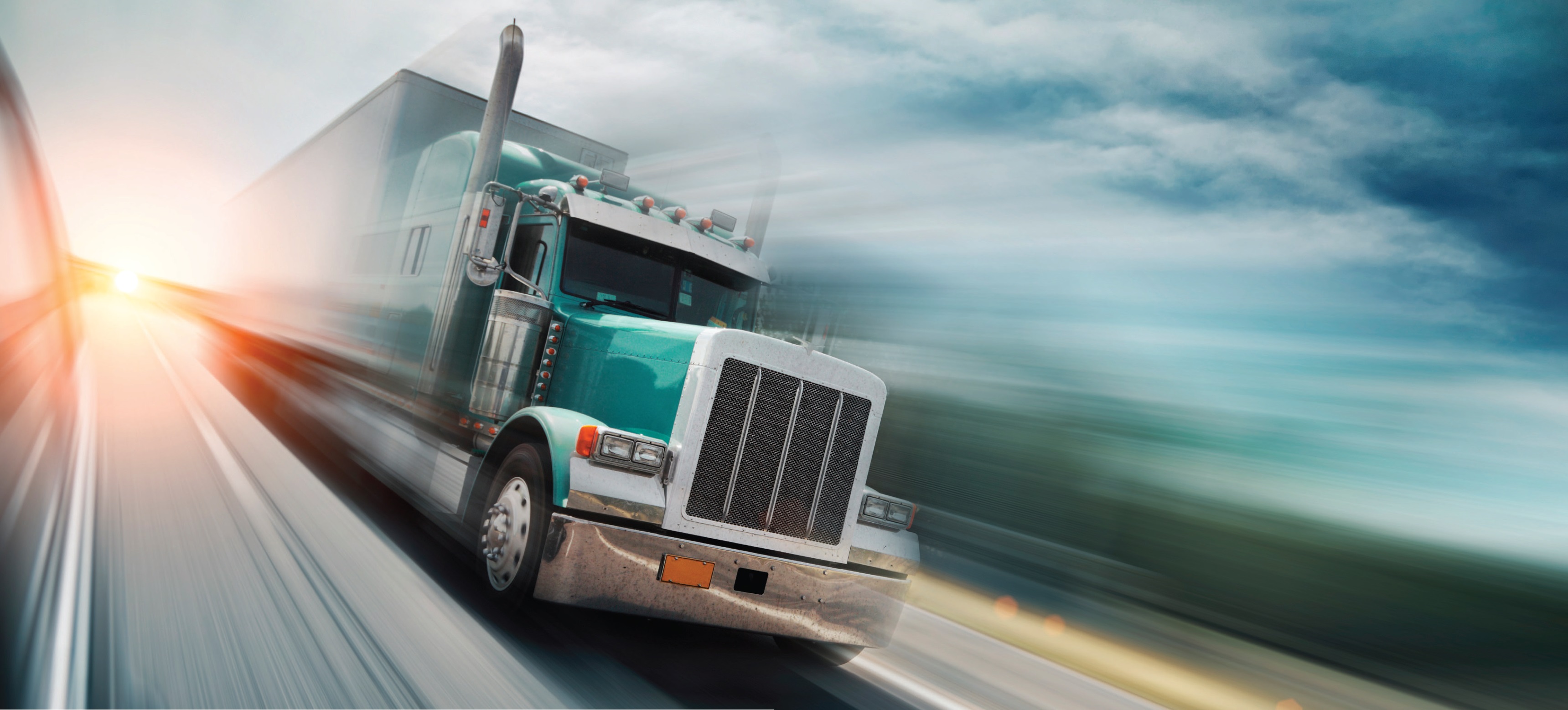
www.canstockphoto.com
KEY CONCEPTS
•
Lubricants have been helping vehicle fuel efficiency since CAFÉ standards were enacted in 1975.
•
The amount of energy manufacturers can save using high-quality lubricants is significant.
•
Cost and lubricant performance are still priorities if you are to provide value to your customers.
MOST LUBRICANTS ARE 70%-90% BASESTOCK (usually mineral oil) and 10%-30% additives. These additives are designed to improve basestock properties such as viscosity and reduce vulnerability to corrosion, friction and wear. Many of those properties, especially viscosity, make a direct contribution to fuel efficiency. Machinery components requiring lubrication that have the greatest affect on fuel efficiency include the crankcase, transmission and gearbox.
“Ever since the U.S. government enacted CAFE (Corporate Average Fuel Economy) in 1975, energy-saving lubricants have significantly helped car and light-duty vehicle manufacturers meet the requirements,” says Jai Bansal, Global Crankcase Technology Advisor for Infineum. “As fuel costs increase, and more stringent fuel economy and/ or greenhouse gas emissions regulations appear on the horizon, vehicle manufacturers are searching with an increasing sense of urgency for fuel economy improvements from every quarter.”
The first standard (18 mpg) did not come out until the 1978 model year (MY). The original idea was to have fuel economy double by 1985—but the actual CAFE rating for the 1985 MY was 27.5 mpg. 2011 was the first model year in 25 years with a target increase above 1985 levels. Ultimately, the 1978 CAFE rating isn’t expected to double until the 2015 MY, meaning it took 40 years to reach a target that was originally 10 years.
While this article refers primarily to liquid fuel efficiency, much of the information applies to the full spectrum of energy sources that power machinery. “Throughout the life of the machine, fuel is one of the largest components of operating costs for our customers,” says Hind Abi-Akar, fluids engineering technical expert for Caterpillar Inc. “Our customers evaluate fuel-saving ideas to assure that they positively contribute to operating profit and that they have no negative impact on machine reliability or durability.”
Fuel-efficient lubricants start with optimized basestock.
LUBRICANT BASESTOCKS
There are a number of ways to categorize basestocks and a number of organizations that have developed their own classifications over the years. For the most part, these classifications are based on the raw material, the level of processing and the chemical structure of the resulting products. In its simplest form, the three types of basestock are as follows:
•
Mineral oils. Mineral basestocks are refined from crude oil by a number of processes. The viscosity of the finished basestock is determined by carbon chain length and processing temperature. Most refiners settle for three or four stocks from which they blend their range of finished oils.
•
VHVIs. Also called unconventional base oils (UCBOs), very high viscosity index basestocks are refined using a two-stage hydrotreating process. VHVIs can be processed to have properties close to those of synthetic oils. Raw material of the VHVI base oil is paraffinic hydrocracking fraction, which is improved by removing waxes through solvent extraction. The raw material is treated with hydrogen under high pressure and at high temperatures in the presence of a catalyst, removing impurities and converting hydrocarbons into paraffinic compounds. The basestock is then stabilized by hydrofinishing. The result is a pure colorless basestock with a stable molecular structure. VHVI basestocks are still mineral oil-based but have unusually high viscosity indexes. They have very low volatility. The use of these basestocks in motor oils is increasing due to their compatibility with lower emission engine components.
•
Synthetics. One of the only commonalities of synthetic basestocks is their chemical process production. Synthetic processes enable molecules to be built from simpler substances to give the precise or desired oil properties required. This group is roughly equivalent to API Group IV. The main disadvantage of synthetics is that they are inherently more expensive than mineral oils. Polyalphaolefins are the most widely used synthetic lubricants in the U.S. and Europe. The main classes of synthetic material used to blend lubricants include:
o
Olefin oligomers: automotive and industrial applications.
o
Dibasic acid esters: automotive and aeronautic applications.
o
Polyol esters: automotive and aeronautic applications.
o
Alkylated aromatics: automotive and industrial applications.
o
Polyalkylene glycols: industrial applications.
o
Phosphate esters: industrial applications.
API has further categorized basestocks by their sulfur content, level of saturates and viscosity index (VI). Following are the five API classifications of basestocks:
•
Group I Solvent dewaxed basestocks. The least refined of all of the groups. They are usually a mix of different hydrocarbon chains with little else in common. These oils are used in applications without high-performance demands.
•
Group II Hydroprocessing and Refining basestocks. Prevalent in commercial mineral-based motor oils. They perform adequately for lubricating properties such as volatility, oxidative stability and flash/fire points, but they leave something to be desired when it comes to cold start viscosity, extreme pressure durability and pour point.
•
Group III Hydroprocessing and Refining basestocks. The most highly refined of all mineral oil basestocks. They perform well in many regards and are highly stable. Lubricants formulated with these basestocks are often labeled
synthetic or
semisynthetic.
www.canstockphoto.com
•
Group IV or PAO (polyalphaolefin) basestocks. Chemically engineered synthetic basestocks. These basestocks have uniform molecular chains and highly stable chemical compositions. These basestocks are increasingly prevalent in synthetic and synthetic-blend products for industrial and vehicular applications. For some applications, they can be significant contributors to fuel efficiency.
•
Group V basestocks. While not often used as stand-alone basestocks, they are used in small quantities as secondary basestocks in special situation to impart certain properties to lubricants. They also provide the raw material for additives. These basestocks are capable of accommodating a wide variety of properties and custom property packages.
“One should not overlook the benefit of high-performance basestocks that can help extend lubricant life,” says Sandy Reid-Peters, performance product specialist for the ExxonMobil Chemical Co. “For the majority of lubricants, the main component is the basestock, typically around 90% or more. As such, the energy savings potential of the lubricant is highly dependent on the ability of the basestock to reduce energy losses.”
www.canstockphoto.com
THE EFFECT OF VISCOSITY ON FUEL EFFICIENCY
Consider that engine oil has the following basic functions:
•
Reduces friction between the moving parts.
•
Transfers heat from the engine to the cooling system.
•
Protects against corrosion.
•
Keeps the engine clean by acting as a solvent for contaminants and isolating particles so that they can be trapped by the oil filter.
•
Creates a contaminate seal between parts.
Low-viscosity oil performs nearly all these functions well, with the added benefit of better fuel economy because the engine is doing less work. “A significant part of the fuel economy gains for hydrodynamic (HD) lubricants accrue from the reduced viscosity profile of the lubricant as opposed to additives that might reduce surface friction,” says Jai Bansal, Global Crankcase Technology Advisor for Infineum.
“Therefore, additives help reduce the viscosity profile of the lubricant such as dispersants, and viscosity modifiers with optimized shear thinning properties are important for HD fuel-efficient lubricants,” Bansal adds. “However, low-viscosity lubricants also carry with them the potential to increase engine wear. Therefore, the additive system must also be designed to ensure that such side effects are minimized in a fully formulated lubricant.”
Hind Abi-Akar, fluids engineering technical expert for Caterpillar Inc., agrees: “The key concern in using low-viscosity oils and oils of thin films in engines is component wear. Bearings, liners and valve trains are of high concern. Other impacts such as on oil pump and engine oil pressure also are of concern.”
“Since there is no experience in using low-viscosity oils, in particular low HTHS oils in HD applications, there is the concern about these oils,” Abi-Akar adds. “Design criteria for engines used in HD applications have been centered on oils of certain HTHS minimum limits. Reducing these limits is of concern due to lack of understanding of the impact and due to the fact that engines so far are designed to use specific HTHS oils reliably.”
According to Reid-Peters, following are the main sources of lubricant-related energy losses on a typical piece of mechanical equipment and an explanation as to how the basestock can help:
1.
Metal-to-metal contact causing friction and energy loss. Metal-to-metal contact occurs when the oil film is not thick enough to completely separate the surfaces. A basestock with a higher viscosity index reduces the likelihood of metal-to-metal contact by maintaining a higher oil viscosity and, thus, thicker oil films at higher temperatures.
2.
Churning and windage of mechanical parts through the oil. Windage and churning results in energy losses due to the hydrodynamic conditions under which the lubricated components are running. The higher the oil viscosity, the more energy that is lost moving the mechanical parts through the oil. In choosing a basestock, it is important to avoid excessive churning and windage losses, especially when the equipment is below normal or typical operating temperatures.
3.
Internal lubricant friction (traction) under elastohydrodynamic (EHD) lubrication conditions. EHD conditions occur when two non-conforming surfaces approach each other with very high contact pressures in a small load zone such as with gears, cams and rolling element bearings. Under these conditions, a thin film of high viscosity oil keeps the surfaces separated. Synthetic basestocks such as PAOs with low traction coefficients offer the ability to save energy, especially where there is a high degree of sliding such as in gears, particularly worm gears.
LUBRICANT ADDITIVES
Once lubricant base oils have gone through typical purifying and quality-improving processes, additives can still significantly impact a host of properties. These additives are often classified based on the following 10 categories of performance:
1.
Detergent additives
2.
Dispersant additives
3.
Oxidation and corrosion inhibitors
4.
Extreme-pressure and antiwear agents
5.
Oiliness agents/friction modifier additives
6.
Antioxidant and metal deactivators
7.
Viscosity Index improvers
8.
Antirust agents
9.
Pour point depressants
10.
Other additives, including antifoam agents.
“Designers of today’s lubricants use a variety of formulation strategies to maximize fuel economy performance of the lubricant,” Bansal says. “Judicious choice of additives is invariably a critical part of these strategies. Not only do the additives have to minimize frictional losses in engine parts that are operating under a broad range of operating conditions, but they also have to ensure that other performance aspects of the lubricants such as wear and cleanliness are not compromised in the effort to maximize the fuel economy gains.”
HOW ADDITIVES WORK
Specific performance-improving additives are geared toward specific base oils. However, the same additive can be effective in more than one class of base oil. Most additives depend on a
competitive reaction mechanism—the additive reacts with an undesirable substance that occurs in the lubrication system that, if unchecked, could lead to premature failure of either the base lubricant or component. For example, when an antioxidant is added to a hydrocarbon lubricant, the additive reacts with the initial free radicals formed early in the oxidation process by delaying or preventing the attack on the base oil.
In general, it’s important that additives are dormant and stable during normal lubricant function and only become active and reactive on demand (when they’re triggered by a specific event). For example, antioxidants in hydrocarbons become active and reactive only when oxidative free radicals are present. Also, an additive may work by more than one mechanism, for example by both blocking and trapping water.
BASESTOCK AND ADDITIVES
Lubricant basestocks influence lubricant performance through two main functions: solubility and oxidative stability. Synthetic base oils exhibit very specific additive solubility that is different from mineral oils. The most common synthetic base oils are synthetic hydrocarbons such as esters and PAOs. Esters vary in additive response and are excellent solvents, except for additives that they react with to form undesirable byproducts. Synthetic hydrocarbons can exhibit excellent additive response (or performance). Some synthetic oils can be blended with each other or with mineral oil to provide the optimum balance of additive solubility and additive response. One of the main reasons for the popularity of synthetic and mineral oil blends is that the additives attached to the mineral oil structure become more soluble with the addition of synthetic oil.
APPLICATIONS
The types of oil lubricants considered, in decreasing order of volume consumed by industry, are as follows (
1):
1.
Circulating oils, including turbine oils
2.
Hydraulic oils
3.
Gear oils
4.
Compressor oils
5.
Lubricating greases.
CIRCULATING OILS AND TURBINE OILS
The following performance requirements are typically required for circulating oils, particularly steam and gas turbine oils:
1.
Provide bearing lubrication
2.
Remove heat through circulation
3.
Serve as hydraulic fluid for control equipment
4.
Lubricate reducing gears
5.
Protect against corrosion
6.
Allow rapid separation of water
7.
Resist foaming
8.
Resist oxidation and sludge formation.
Following are types of additives typically used in circulating oils and turbine oils:
•
Antioxidants. Used to inhibit the attack of turbine oil by oxygen and to reduce the formation of undesirable oxidation products like corrosive organic acids and insoluble sludge.
•
Rust inhibitors. Turbine oils are formulated to prevent rust in areas in contact with the lubricant.
•
Metal deactivators. Prevent corrosion of copper and copper alloys such as bronze and brass.
•
Foam inhibitors. Usually added to turbine oils.
•
Demulsifiers. Separate water from oil.
The following performance requirements are typically required for hydraulic oils:
1.
Long life and consistent performance
2.
Optimal viscosity and compressibility
3.
Corrosion/oxidation protection
4.
Wear protection
5.
Thermal stability
6.
Hydrolytic stability
7.
Filterability
8.
Demulsibility/emulsibility
9.
Compatibility with system components.
Following are types of additives typically used in hydraulic oils:
•
Antioxidants. Hydraulic oils, for example, may use organometallic antioxidants that act as antiwear additives and secondary antioxidants.
•
Rust inhibitors. Used in hydraulic oils that might contain metals, such as metal sulfonates, and which might have a higher than usual proportion of base to neutralize acidic oxidation products.
•
Antiwear additives. These are needed to inhibit frictional wear of moving parts.
•
Metal deactivators. Because of the possible corrosiveness of the decomposition byproducts of antiwear additives, an effective metal deactivator is crucial in antiwear hydraulic oils.
•
Foam inhibitors. Used to prevent foam or entrained air from interfering with the power transfer function of hydraulic oil.
•
Viscosity Index improvers. Used to increase the operating temperature range of hydraulic oils.
•
Pour point depressants. These lower the pour point and allow mineral and hydraulic oils to be pumped at low temperatures.
IL-SAC GF-5 SPECIFICATON (4)
The ILSAC GF-5 Specification was ratified in December 2009 and introduced to the market in October 2010. The GF-5 specification provides the following three new improvements over GF-4:
•
Emission Compatibility: Seq IIIG Phosphorus retention
•
Oil Robustness: Seq IIIG WPD, TEOST MHT and 33C, E85 emulsion
•
Fuel Economy: Seq VI-D.
GF-5 provides the following means of achieving the three improvements:
•
Emission Compatibility: Seq IIIG-B at 79% min. phosphorus retention
•
Oil Robustness: Seq IIIG WPD of 4.0 min, TEOST MHT 35 max
•
New Fuel Economy: Seq VID limits.
ILSAC GF-5 represents the latest performance standard set by the International Lubricant Standardization and Approval Committee. Most automobile manufacturers are expected to recommend oils that meet ILSAC GF-5 in the near future (if they aren’t already).
GEAR OIL ADDITIVES
Gear oil performance requirements can vary substantially depending on the amount of sliding and rolling and the load-carrying limits of gear types.
Efficient gearboxes yield higher gear speeds and loads and higher oil operating temperatures. To that end, base lubricants and additives should provide:
1.
A purer product
2.
Improved thermal stability
3.
Better high-temperature performance
4.
Better oxidative resistance
5.
Longer demulsibility time
6.
Lower friction
7.
Better surface fatigue protection
8.
Better foam resistance.
Following are additives typically used in gear oils:
•
Extreme pressure additives. Organic sulfur and phosphorous compounds and inorganic borates are primary components in gear oil additives.
•
Friction modifiers. Modifiers such as acidless tallow may be used in applications like worm gears that involve sliding contact.
•
Viscosity Index improvers. Since gear oils often encounter greater mechanical shear than hydraulic oils, polymers used in VI improvers in gear oils must have exceptional shear stability.
•
Antimisting additives. In order to protect workers, care must be taken to minimize stray mist.
FULL API SN CERTIFICATION (5, 6)
The API Lubricants Group finalized the user language and test requirements for both API SN and its supplemental category, Resource Conserving. This new supplemental category expands the previous Energy Conserving supplemental category (which only refers to fuel economy claims) by:
•
Adding greater emission system protection
•
Adding turbocharger protection
•
Enabling engines to operate on ethanol-containing fuels up to E85.
While API SN, when licensed with Resource Conserving, is equivalent to ILSAC GF-5, SN alone does not require:
•
Sequence VID Fuel Economy
•
Sequence IIIGB Phosphorous Retention
•
TEOST 33C
•
Emulsion Retention.
The first allowable license date for API SN coincided with the ILSAC GF-5 first license date of Oct. 1, 2010. Details of the SN engine oil performance standards are given in
Technical Bulletin 1— API 1509, Engine Oil Licensing and Certification System.16th Edition, April 2007.
COMPRESSOR OIL ADDITIVES
Similar to gear oils, performance requirements can vary substantially. Requirements depend on such things as compressor type, the properties of the gas being compressed and discharge pressures and temperatures. A significant portion of compressor oils have a synthetic base.
Universal lubrication requirements for compressor oils include:
1.
Low flammability
2.
Oxidation resistance
3.
Thermal stability
4.
Viscosity retention
5.
Antiwear capabilities
6.
Corrosion prevention
7.
Good demulsibility.
GREASE ADDITIVES
In addition to a lubrication base and additives, lubricating greases also include thickeners. Therefore, it’s important that additives are compatible with the thickener.
Following are types of additives typically used in lubricating greases:
•
Rust and oxidation inhibitors. Greases for most applications generally require only antiwear additives and rust and oxidation inhibitors.
•
Antiwear and extreme-pressure additives. EP and antiwear additives are used with heavier loads where the lubricant alone may not prevent metal-to-metal contact.
•
Polymers. Where water is present (for example, paper mills), polymer additives are highly desirable.
•
Grease thickeners. Specialized formulations perform double-duty as both grease thickeners and performance additives.
REGULATIONS AND CERTIFICATIONS
In 2010 the EPA and the National Highway Traffic Safety Administration (NHTSA) introduced new initiatives to reduce greenhouse gases and improve fuel efficiency from on-road vehicles and engines. “These initiatives include developing first-ever greenhouse gas regulations for heavy-duty engines and vehicles,” Bansal says. “In response to these regulations, the lubricant industry has begun the process of defining the next generation of hydrodynamic (HD) lubricants. Fuel economy performance is expected to be a key feature of this new generation of lubricants.”
IL SAC GF-5
Interested parties such as lubricant additive industries and oil marketers are working to develop and define the International Lubricant Standardization and Approval Committee’s ILSAC GF-5. The purpose of these GF-5 oils will be to improve the function and performance of cutting-edge engine and emission designs while at the same time facilitating compliance with the new government fuel economy and emission regulations.
API SN
The service rating of motor oils is classified by API with the familiar API “Service Symbol Donut” on the product label. SN is the newest service category rating for gasoline engines in 2011 cars and light trucks. SN engine oils are designated “resource conserving” because they help improve fuel economy and protect vehicle emission system components. Additionally, these oils have demonstrated in tests that they provide greater emission system and turbocharger protection and help protect engines running on ethanol fuels. The API SN rating is roughly equivalent to the GF-5 oil rating.
WHERE TO START
In addition to reducing energy bills, upgrading lubricants also reduces maintenance costs, replacement costs and pollution (both inside and outside the facility). So the costs for the machine itself, its maintenance and lubricant consumption all go down.
Following are averages of documented, repeatable energy savings achieved based on one company’s customer readings after upgrading the following equipment to a more energy-efficient lubricant (
2):
•
Drive chains/conveyors: from approximately 20% at startup to a running amperage reduction of up to 26%
•
Hydraulics: power consumption cut by up to 7%
•
Gearboxes: from 6%-12% with exceptions up to 50%
•
Engines: averaging 5%-6%.
The amount of energy that manufacturers can save using high-quality lubricants is generally between 5% and 15%. The actual savings can be greater, but it depends on factors such as the type of equipment and how it’s used. While lubricants can be upgraded during a scheduled service, they can also be enhanced by adding energy-saving oil additives in the interval. It makes sense to start using energy-saving lubricants with machines that use the most energy.
“Off-road construction and industrial equipment operating in heavy-duty applications include high loads and a myriad of cycles that vary per machine type and application,” Abi-Akar says. “We must ensure that lubricants protect the engine-moving components under high loads, allow the machine to reach its designed life and preserve the resale value of the machine as well as support machine reliability.
“At the same time, these lubricants must have an overall positive financial value to the customer. If any of the above is potentially compromised due to oil performance, or if fuel savings are difficult to measure, customers typically choose not to put their operations at risk by using unfamiliar consumables (including lubricants),” says Abi-Akar.
Chain conveyor systems and gearboxes are prime beneficiaries of improved lubricants. Screw-type compressors and hydraulic installations also tend to take up much of the industrial energy use. High-quality lubricants can lower the energy expended in these installations by about 10%.
GEARBOXES
Gearboxes can be inefficient energy users—this is especially true for gearboxes with worm gears. Significant energy savings can nearly always be realized in gearboxes by reducing the friction.
Saving energy in gearboxes can be accomplished with oil additives, high-quality oils and semifluid greases. Using infrared temperature measurements, the effect of less friction through higher-quality lubricants in a gearbox is easily proved.
Chains and Conveyor Belts. Savings are realized with dry, dirt-repelling lubricants. Because of the relatively high lubrication frequency and wear in chains and conveyor belts, it is nearly always profitable to switch to a better lubricant. Savings increase with use intensity and load weight.
Compressed-Air Systems. In typical compressed-air systems, more than 90% of the energy expended is converted to heat and lost. Air leak is often the cause, accounting for up to 40% of energy waste. An easy solution is often lowering the air pressure. But the addition of oil additives to the compressor oil in the fan block nearly always lowers the energy used by the compressor.
Hydraulics. Hydraulic systems use oil to transfer force, usually with oil, so the oil viscosity is selected by primarily taking into account the optimal reaction time of the system, not the optimal lubrication. Improving the lubrication capacity of the hydraulic oil significantly reduces friction. The result is that oil temperatures and, therefore, energy use go down and oil life increases. Because of this, adding oil additives to hydraulic installations can quickly achieve major savings.
Fans. Vibrations resulting from high friction cause energy loss in fans. This creates unnecessarily high bearing temperatures. Since fans are often difficult to access, reducing the need for lubrication servicing greatly reduces maintenance costs.
Water pumps. The cost for the energy and maintenance of water pumps is high when compared to other types of machinery. Because of the labor-intensive nature of lubricating water pumps, lubrication with high-quality, corrosion-proof lubricants with significantly longer service intervals saves money.
For passenger cars, there has been a general trend toward lighter viscosity grades of lubricants along with friction-modifier additives. Engine oils can contribute to improvements in fuel economy in two ways: (1.) Indirectly, by supporting engine design that allows fuel economy without compromising high performance and (2.) Directly, through new formulations that improve fuel economy. Both routes require the right balance of base oil and additives.
TESTING
“Measuring energy savings is very challenging for heavy-duty applications,” Abi-Akar says. “Engine efficiency alone is not the key contributor to non-road fuel efficiency, and at this time we don’t believe that there is a test or tools to accurately measure energy efficiency in non-road equipment in a repeatable way—in particular lubricant-based efficiencies—and relate them to actual field applications. The variety of duty cycles of various machines and applications does not lend itself to one method of measuring energy savings. Field tests are lengthy and prone to lack of control. We will continue to work with the industry to develop tools that measure efficiency.”
Reid-Peters doesn’t completely agree: “Industry has already made some needed improvements. For instance, the sequence VIA engine oil test, used for many years to evaluate fuel economy, only evaluated the ‘fresh oil’ fuel economy. The sequence VIB, and more recently the sequence VID engine tests, now check the fuel economy on both fresh oil and used oil, using a more modern and more efficient engine. This will provide for better measurement of efficiency gains over the lifecycle of the motor oil. The tools for measuring energy savings have become better but, as overall equipment efficiency improves, new incremental energy savings are smaller and more difficult to measure.”
Adding oil additives to hydraulic installations can quickly achieve major savings.
www.canstockphoto.com
SEQUENCE VID TEST FOR VEHICLES
The Sequence VID is an engine dynamometer test that measures a lubricant’s ability to improve the fuel economy of passenger cars and light-duty trucks. The VID method compares the performance of a test lubricant to the performance of a baseline lubricant over six different stages of operation.
For example, a 2008 Cadillac SRX 3.6L High Feature (HF) V6, 4-cycle engine. The engine includes dual overhead camshafts, 4 valves/cylinders, a dual-stage plenum induction manifold, a 94x85.6mm bore and stroke, with a 10.2:1 compression ratio.
The Sequence VID test incorporates a flush and run procedure. Each test consists of two 6-stage fuel economy measurements on baseline oil (BL), one at the beginning of the test and one at the end.
The test oil is evaluated in-between the two baseline runs. The test oil is initially aged during 16 hours of engine operation at 2,250 r/min and 120 C oil temperature. After the initial aging, a 6-stage fuel economy measurement is taken. The test oil is then aged an additional 84 hours at an engine speed of 2,250 r/min and 120 C oil temperature.
Following this final aging, the test oil once again goes through a 6-stage fuel economy measurement. The two fuel economy measurements taken on the baseline oil and a final value for fuel economy improvement is calculated for the test oil (
3).
Vehicle manufacturers are searching with an increasing sense of urgency for fuel economy improvements from every quarter.
www.canstockphoto.com
THE FUTURE
Based on market trends and feedback from their customers, ExxonMobil recently developed and introduced SpectraSyn Elite,™ a new polyalphaolefin (PAO) based on metallocene catalyst technology which offers higher viscosity indices, better low-temperature fluidity and better shear stability than conventional PAOs. “ExxonMobil Chemical is continually investing in new technology designed to improve lubricant performance,” says Reid-Peters.
New lubricant standards eventually will require oils to provide improved fuel economy and include additive chemistry that does not degrade emission system components. Because of increasing cost and dwindling energy sources, the opportunity for significant savings (particularly in rotating equipment) through improved lubrication base oils and additives is increasing.
“We are creating machine fuel consumption improvements through implementation of engine combustion efficiency improvement, more efficient machine systems—hydraulics, more efficient transmission/driveline technologies and, most important, by integrating multiple machine systems,” Abi-Akar says. “No specific approach is a panacea for saving energy. We continue to pursue multiple technologies and worksite management concepts designed to reduce operating cost and positively contribute to our customers’ bottom line.”
While energy cost is in the operating budget and service is in the maintenance budget, tracking energy savings that results from improved lubrication creates the opportunity to reduce maintenance costs and increase overall return-on-investment for equipment. With high-use, high-energy-using equipment, the switch to an energy-saving lubricant can start paying for itself quickly (high-quality lubricants last up to five times longer).
“Energy-saving lubricants is an important area of research at Infineum,” Bansal says. “Our scientists are working on a variety of approaches to enhance our ability to offer leading edge lubricant solutions to our customers.” Some of the key aspects of Infineum’s R&D activity include:
•
Quantification of lubricant-accessible energy losses in the engine and how they are distributed over various parts of the engine.
•
Development of additive components and formulation strategies to minimize the internal frictional losses in different engine parts operating under different tribological regimes, as well as to mitigate engine wear that may occur as the lubricant viscosity is minimized in an effort to further increase the energy conserving capability of lubricants.
•
Innovative approaches to demonstration of lubricant fuel economy performance under real-world conditions.
“In the grand scheme of things, the fuel economy improvements that can be gained from engine lubricants might seem relatively small,” Bansal says. “But in today’s tough market, they are becoming increasingly important.”
REFERENCES
1.
From
here.
2.
Interflon (from
here).
3.
Intertek (from
here).
4.
From
here.
5.
From here.
6.
Also from
here.
Jean Van Rensselar heads her own communication/public relations firm, Smart PR Communications, in Naperville, Ill. You can reach her at jean@smartprcommunications.com.