What is ‘green?’
Dr. Neil Canter, Contributing Editor | TLT Feature Article December 2010
Working without a commonly agreed upon definition, lubricant manufacturers are creating products to protect both the environment and machinery.
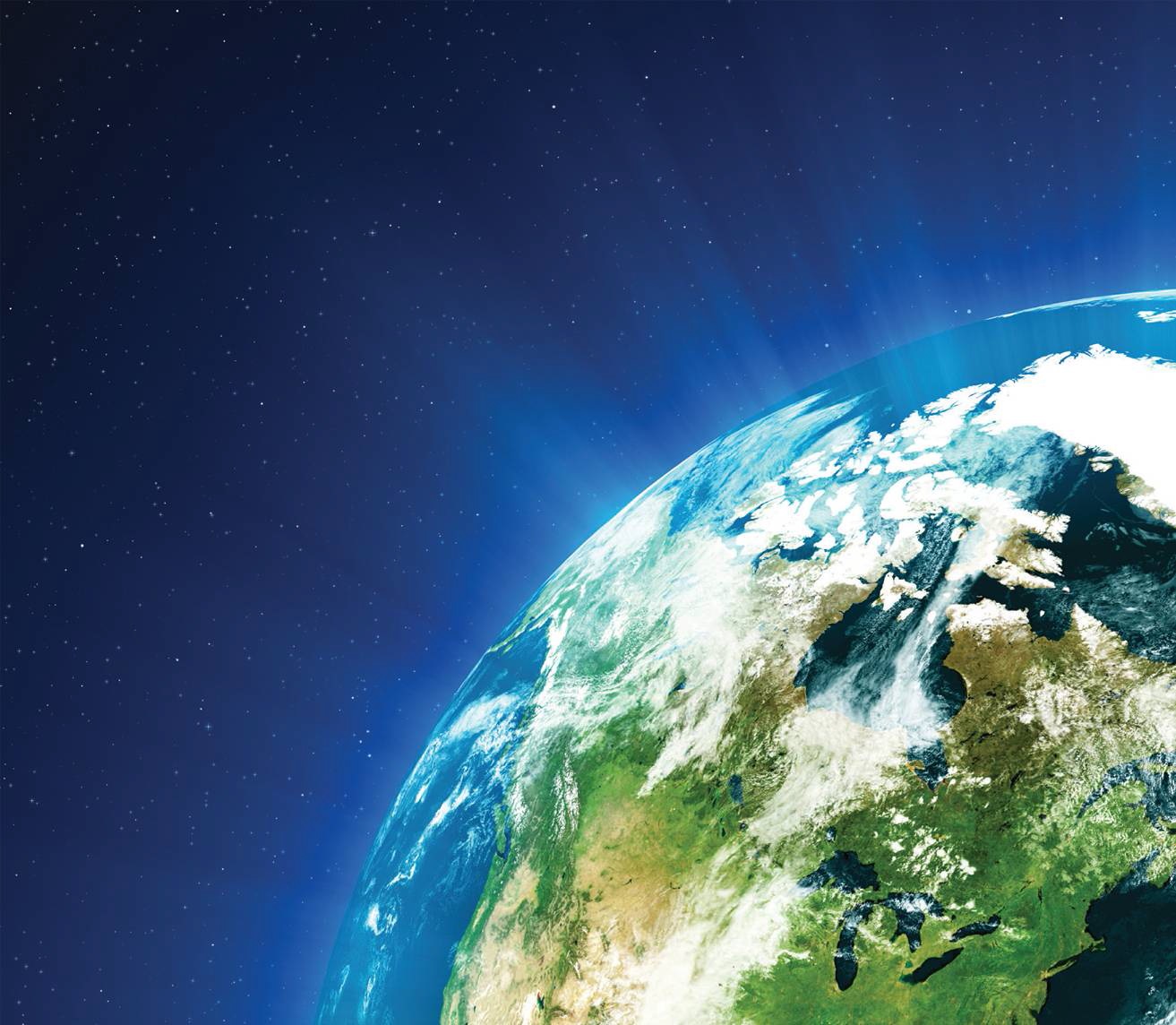
www.canstockphoto.com
KEY CONCEPTS
•
Although associated with environmental protection, the terms ‘green’ and ‘sustainable’ have no universal definition or commonly agreed upon standard.
•
The answer to “what is green?” is complex and based on development of products that reduce the use of hazardous substances, minimize pollution of the environment, gain social acceptance and are still economically viable.
•
A new standard is in the offering to help end-users understand the sustainability of specific chemical substances and their manufacturing process.
The term ‘green’ is receiving an increasing amount of attention because it has become associated with biodegradable lubricants claimed to be derived from natural or renewable sources and which degrade at a specific rate. A second term, sustainability, also has entered the discussion.
While the question raised is a simple one, the answer is much more complex and has not even been clearly made. Much of the focus on green has been to determine the raw material sourcing and final disposition of a lubricant. These factors are important, but what is missing is how the basic functions of a lubricant impact the actual application. After all, lubricants are designed to boost productivity and reduce energy use. These parameters must be considered in providing answers to the “what is green” question.
This article is an update on the progress being made to define a green, sustainable substance or process. Examples are provided for lubricants that go beyond meeting just the basic biodegradable criteria and actually improve the efficiency and energy savings of a specific process compared to existing technologies.
MANY DEFINITIONS
With all the attention paid to raw materials, guidance in defining green and sustainability can be found by looking to the chemical industry. The ACS Green Chemistry Institute® (ACS GCI) was established in 1997 to provide more information and awareness about green chemistry and now is part of the American Chemical Society.
Dr. Jennifer Young, manager at ACS GCI in Washington, D. C., says, “Green chemistry is the design of chemical products and processes that reduce the use and generation of hazardous substances. This definition can be applied across the chemical enterprise, including the chemical manufacturer and formulator.”
Andy Shafer, executive vice president, sales and market development, for Elevance Renewable Sciences in Bolingbrook, Ill., differentiates between green and sustainability. He says, “A substance is defined as ‘green’ based on the degree to which the feedstock is biobased vs. petroleum-based, how much energy is used during the manufacturing process or life cycle and the impact the substance has to people and the environment. In contrast, sustainability has economic, environmental and social aspects.”
Economics is the initial consideration because products are selected on a cost-use basis. Second, the environment must be considered. In addition to having an acceptable level of performance, products cannot be toxic, pollute the environment or lead to disposal problems.
Finally, the social category involves the willingness of people to accept the use of the product. Shafer elaborates, “The objective of all people around the world is to lead productive lives. People are evaluating whether a specific product or technology is going to help them better their lives and enable future generations to have the same opportunities.”
STLE-member Chris Donaghy, director of lubricants and polymer additives for CRODA, Inc., in New Castle, Del., says, “Green chemistry is the design, development and implementation of chemical products and processes to reduce or eliminate the use and generation of substances hazardous to human health and the environment.” He indicates the challenge in the marketplace is that there is no universal definition, which means that his company relies on the input of lubricant suppliers to develop green products that meet their needs.
Sustainability is concerned with how a product is manufactured with regard to the environment. Donaghy says, “A sustainable substance should be based on resources that will not be exhausted over a period of reasonable time and it should not generate unacceptable pollution, both internally and externally.”
STLE-member Neil Higgins, research fellow, R&D for Bunge North America in Bradley, Ill., says, “A green product is one that has the least environmental impact across its life cycle. Entropy wins, so every process or product extracts a toll on the environment.”
Higgins agrees with the previous definitions on sustainability and provides further insight into how humanity needs to make changes to ensure future generations lead productive lives. He says, “Sustainability is the ability to make a green product over generations with the lowest toll on the environment. Rice produced on terrace in Asia has been maintained for over 2,000 years and is an example of a sustainable enterprise. Industrialization has greatly enriched humanity but has to date been carried out in a manner that is not sustainable. The processes to produce the bounty of industrialization must be re-engineered to take a lower toll in terms of energy, water and land used per unit of goods produced.”
Jim Murphy, product manager-Hot Mill Lubricants for Quaker Chemical Corp. in Conshohocken, Pa., says, “A green substance exhibits a positive impact on the environment. The effect of the substance can be determined by measuring its carbon footprint.”
Carbon footprint measures the amount of greenhouse gases emitted by a specific substance used in a particular process. It is measured in a positive, neutral and negative fashion. The former means that a process reduces the emission of greenhouse gases by taking out more gases than it emits. A neutral carbon footprint indicates no change while a negative rating reflects an increase in emissions.
Murphy indicates that sustainability reflects a process that maintains a positive carbon footprint. The carbon footprint is further segmented into primary and secondary categories. The former refers to the use of energy captured from natural resources that can include crude oil, coal and natural gas as well as all the energy necessary to produce a product by use of those resources. The secondary carbon footprint refers to the transformation of primary or secondary energy sources in an industrial process.
John Cullinane, president of LiQuifix Inc. in Stamford, Conn., says, “A green or sustainable substance is one that is minimally harmful to the environment or to humans in its production, use and disposal.”
A GREENER STANDARD
Young indicates that ACS GCI is working with industry stakeholders to develop an information standard to allow end-users to assess the sustainability of specific chemical substances and the manufacturing processes by which they are made. This is the first standard to incorporate both chemical and chemistry information. She says, “The Standard for Greener Chemicals and Processes Information will involve providing a list of chemical characteristics and processes information.”
The first aspect of a greener chemical involves looking at such issues as its physical properties, including reactivity, (For example: Is it explosive and does it react with water?), human effects (irritation, toxicity, etc.) and ecological properties (persistence, degradation and aquatic toxicity).
The process side of the definition evaluates how the company manufactures a specific chemical substance. Says Young: “From the process standpoint, we are looking to see how much energy is used in terms of carbon dioxide equivalents and how efficient is the procedure. The latter is determined from the ratio of how much raw material is inputted to how much finished product is obtained. Recycling of components used in the process also is accounted for in calculating the efficiency.”
Figure 1 is a schematic showing the processing of raw materials into chemical intermediates and then finished products such as lubricants. All these elements need to be analyzed to determine if a specific finished product is worthy of being classified as a greener alternative.
Figure 1. An information standard under development will enable users to assess the sustainability of specific chemical substances from extraction until their end- of-life phase, as noted in this chart. (Courtesy of The ACS Green Chemistry Institute®)
“We feel that the standard should be focused on individual chemical substances instead of formulations because it was written with chemical manufacturers as the primary users of the standard,” Young says. “The idea is to have the user make a more informed decision about whether to use one specific chemical substance as compared to another in a specific application. We decided not to provide any limits or values so users will need to use the standard on a comparative basis.”
The proposed new standard will be administered by NSF International and is known as NSF/GCI 355. Young reports that a draft was recently published in mid-September and was under review by interested parties through mid-November.
LUBRICANT IMPACT
The choice of the proper lubricant can have a major effect on the sustainability of a specific manufacturing process. In particular, measuring the carbon footprint is a quantitative way of determining the capability of a green lubricant.
Donaghy says, “Carbon footprint is an objective way of measuring the sustainability of a product from ‘cradle to grave.’ We run carbon footprint analysis on many of our products using PAS 2050.”
PAS 2050 is a method derived by the BSI British Standards to measure greenhouse gas emissions (
1). The technique relies on a life cycle assessment.
The environmental impact of a lubricant life cycle is shown in Figure 2. Donaghy says, “Life cycle analysis is an assessment of raw material production, manufacture, distribution, use and disposal. It also should include all transportation steps necessary for supplying a product.”
Figure 2. The factors shown in this chart need to be considered in evaluating the life cycle of a lubricant. (Courtesy of CRODA, Inc.)
Figure 2 depicts factors that need to be considered in a lubricant’s life cycle: manufacturing, stability, performance and dealing with the lubricant when it reaches the end of its operating life. For the manufacturing phase, questions about the renewability of the process and its efficiency must be answered. Lubricant performance is an extremely critical aspect because its ability to reduce friction and wear can be quantified into energy savings.
Stability is a measure of how long a lubricant can perform at an optimal level under use conditions. The point being made is that an end-user needs less of a longer-lasting lubricant.
At the end of its operating life, questions to answer include: Can the lubricant be readily biodegradable, can it be recycled into a material that is useful and can energy recovery be realized through combustion?
Steve DiBiase, chief science officer for Elevance Renewable Sciences in Bolingbrook, Ill., addresses the issues of a lubricant’s life cycle and carbon footprint. He says, “From a life cycle perspective, the important issue is how the lubricant performs during use. The green nature of a lubricant can be measured by its reduction of energy use through fuel economy of engine oils or power consumption during manufacturing and use. In addition, how effective a lubricant is in extending the operating life of manufacturing equipment or how long the interval between lubricant changes is very important to determining its performance.”
The latter directly affects the cost of lubricant disposal or recycling during its end-of-use phase. DiBiase believes that the composition of the lubricant should not be ignored in determining its green profile. He says, “The components used in formulating a specific lubricant need to be evaluated for such issues as biodegradability and toxicity. Formulations using high amounts of components such as heavy metals and chlorine contribute to this issue.”
Coupled with this is a consideration of the lubricant application. DiBiase says, “The degree of lubricant exposure to the environment is an important parameter. For example, a lubricant used in a chainsaw will undoubtedly be lost to the environment, while motor oil has minimal exposure outside of the engine.”
All of these issues lead to the social acceptance of the lubricant, a driving force in its success. Shafer adds, “Product safety and worker exposure are among the top considerations taken into account to determine if the lubricant is socially acceptable.”
Another consideration for using green lubricants is the impact of volatile organic compounds (VOCs). There is a general trend moving toward low-VOC-containing substances.
STLE-member Frank Lochel, senior chemist for Cargill Corp. in Wayzata, Minn., says, “The need for low VOCs is highlighted by a recent new standard to measure VOCs that has been adopted by the Southern California Air Quality Management District (
2). It establishes VOC limits for metalworking, metal treating, metal-protecting fluids and vanishing oils. VOC values will be measured by a new analytical technique based on thermogravimetric analysis. The method is in a standard designated as ASTM E1868-10.”
Lochel believes that the low-VOC characteristics of green lubricants will lead to their wider use because they provide more efficient machining and lower stack emissions in applications where lubricants are taken to elevated temperatures. Both benefits contribute to large energy savings.
RANKING LUBRICANT BENEFITS
Such benefits as energy savings, environmental benefits, friction and wear reduction are used to evaluate the sustainability of a lubricant. The challenge is finding a way to rank them.
Donaghy says, “These parameters can be ranked for the overall lubricant industry but need to be evaluated on an application-by-application basis. For instance, for a PCMO application, friction reduction has a much more dramatic effect on the environment than wear reduction (when was the last time you heard of an engine wearing out–typically something else on the car goes way before that).
For other applications (i.e., metal cutting), the wear of tool parts might have a more dramatic effect on the sustainability of the process if the lubricant delivers performance that dramatically improves the tool life, thereby reducing the need to manufacture additional parts. Energy is also required to recycle those parts not meeting a minimum quality control level.”
DiBiase sees evaluation of lubricant benefits as a process that starts with looking at a piece of equipment and determining how to get every bit of efficiency and cost out of the inputted energy. He says, “There is no real reason to rank parameters, as all can be very important to the process. For instance, if you ask someone if they want either clean air or clean water, obviously the answer is both. The idea is to evaluate the process and improve specific parameters to continually improve.”
Shafer considers this an ongoing process to develop better products. He says, “Society as a whole has used petroleum-based products over the past 100 to 200 years. Now we are faced with a more complex situation where fuel and feedstock sources are scarce, and prices are increasing. We have options that are not only petroleum-derived but are available from alternative sources. The objective remains that price, performance and sustainability are all necessary as we consider sources of fuels and feedstocks.”
‘A lubricant used in a chainsaw will undoubtedly be lost to the environment, while motor oil has minimal exposure outside of the engine.’
BIOREFINERIES
One approach to improving sustainability is developing a process for manufacturing lubricants using renewable raw materials. This is the principle behind a biorefinery, which operates in a similar fashion to a conventional petroleum refinery.
Instead of fractionating crude petroleum oil into the various product streams used to manufacture base oils, fuels and petrochemicals, a biorefinery operates in a comparable fashion using renewable raw materials. An example of a biorefinery is the use of renewable feedstocks to prepare internal and alpha olefins, oleochemicals and specialty chemicals such as esters. Many of these types of products are already derived from petroleum-based raw materials and are commonly used in the marketplace.
Shafer says, “A successful biorefinery must make economic sense. Products must be manufactured with good cost profiles and perform at levels that are at least equivalent if not better than petroleum-based chemicals. It is also important that the biorefinery utilize low temperature and low pressure process conditions to leave a low energy footprint.”
Multiple feedstocks need to be utilized to ensure good economics and sustainability. One way to do this is by establishing biorefineries in multiple locations around the world. For example, palm oil is a suitable feedstock for Southeast Asia while soybean oil is better situated for use in North and South America.
Shafer indicates that his company will be starting up a biorefinery during the second quarter of 2011. Annual capacity is 180,000 metric tons or 400 million lbs.
ENERGY RATIO
The sustainability of lubricants also can be measured through determining the energy ratio. This term describes the ratio between how much energy is generated during the service life of a lubricant and the energy required for its manufacture.
Figure 3 shows a chart comparing the energy throughput for a compressor, hydraulic pump and an automobile compared to the energy needed to manufacture synthetic basestocks, mineral oils and vegetable oils. Donaghy says, “The energy throughput for a lubricant application is at least two orders of magnitude higher than for the manufacture of one of the lubricant basestocks shown. If a lubricant containing one of the synthetic basestocks displays even a 1% energy efficiency savings, then this graph suggests that the improvement seen will more than pay back for the additional energy required to manufacture the basestock.”
Figure 3. The energy ratios of lubricant applications such as compressors are far greater than those for the manufacture of mineral oil, synthetic and vegetable oil basestocks. (Courtesy of CRODA, Inc.)
The higher energy costs for manufacturing synthetic basestocks are not surprising because multiple processing steps are needed to produce species such as esters. In that case, high operating temperatures can add considerable energy costs. But Donaghy maintains that the added performance benefits of synthetic basestocks should lead to energy savings. “Energy efficiency claims are always contentious,” he says, “but in most applications energy efficiency improvements of approximately 5% can be realized over time because of optimized lubrication through synthetic oil.”
GREEN HOT MILL LUBRICANT
The lubricant industry is learning how to apply the concepts of green and sustainability toward its applications. An interesting example involves development of a new technology for use in the hot mill section of a steel mill, a major energy consumer. This technology has been found to reduce both the primary and secondary carbon footprint in steel mills.
Murphy says, “Prior to 1990, the U.S. steel industry was very inefficient. Since then, the industry has taken matters into its own hands and improved productivity, which has included significantly reducing its carbon footprint.”
Most of the energy used in a hot mill is needed to melt the steel. Murphy says, “Based on a white paper published by John Stubbles, 80% of the total energy needed to process steel is needed in the steelmaking step (
3). Ernest Orland Lawrence estimates that the amount of energy needed to operate a hot mill is between 500 and 667 kilowatt hours per metric ton (
4). In 2000, a U.S. Department of Energy report indicates that one metric ton of steel produced in a hot mill leads to the generation of 783 pounds of carbon based on energy use.”
With much of the efficiency improvements realized in the steelmaking segment, Murphy maintains there is ample opportunity to improve the efficiency of hot mill rolling, which he judges accounts for between 10% and 15% of the energy use.
A change in the type of hot mill steel lubricant can have a significant impact on steel production. Murphy says, “Steel is heated up to 1,090 C in a furnace and then reduced in thickness by one to two inches per gauge in hot mill processing. A lubricant concentrate is mixed with water at a ratio of 1:100 to facilitate this process in a technique called roll bite lubrication.”
Of the four functions of a hot mill lubricant, Murphy believes that extending the life of the roll is the most important. Other functions include improving the quality of steel products, energy reduction and productivity. The quality issue also impacts latter steps in the steel manufacturing process. Murphy adds, “If scale can be prevented from forming on the steel surface, then procedures used in the manufacture of steel coil such as pickling can be accelerated, reducing energy use.”
An improvement in the type of hot mill lubricant used can have an enormous impact on energy use. Murphy estimates that for every 1% reduction in energy used in a hot mill, the carbon footprint declines by 7.83 pounds per ton. This means that a mill manufacturing 3 million tons of steel per year can realize nearly a 23.5 million pound reduction in its carbon footprint on an annual basis.
Murphy reported on the evaluation of two lubricants prepared from sustainable raw materials compared to a conventional hot mill lubricant containing petroleum oil and also formulated with extreme pressure/antiwear additives (
5). Lab testing displayed significantly lower coefficient of friction values using a high-temperature reciprocating wear instrument. At a temperature of 315 C and with water having a coefficient of friction of 1.00, the green hot mill lubricants exhibited values between 0.08 and 0.12. In contrast, currently used hot mill lubricants have coefficient of friction values between 0.40 and 0.88.
Antiwear testing was conducted on a modified Falex Vee Block and Journal machine. The test measures the amount of metal removed from a test pin, which simulates a steel roll. Conventional hot mill lubricant technology caused as much as 300 milligrams of metal to be removed from the pin. The new green hot mill lubricants reduce wear loss well below 50 milligrams.
Field testing in actual hot mill applications demonstrated the effectiveness of the new green lubricant technology in reducing energy use. Most steel mills have an advanced computer system that measures the force and amperage used by motors doing the actual operations, according to Murphy.
Using water as a reference, the new green hot mill lubricants exhibited on average a 21.7% reduction in force while using 88% less volume than water. Amperage was reduced by 5.8% vs. water.
In contrast, conventional hot mill lubricants display a lower force reduction of 4.6% and amperage drop of 5.8% as compared to water. Figure 4 shows images of rolls lubricated with a green hot mill lubricant vs. just water. The lubricated roll in the left image displays a uniform surface. In contrast, lubrication with water either produces oxide bands in the center image or tearing and roughing in the right image. The latter photo shows how the lack of lubrication can lead metal to peel off of a roll, which is an indication of heavy wear.
Figure 4. A green hot mill lubricant displays superior performance, as noted in the left image, compared to the use of water in the other two images while providing the added benefit of further reducing the carbon footprint of the application. (Courtesy of Quaker Chemical Corp.)
Murphy estimates that a significant carbon footprint reduction can be achieved in using the green hot mill lubricant. He says, “Based on empirical calculations, the carbon footprint of a green hot mill lubricant can be reduced as compared to water by 15% per ton. In contrast, a conventional hot mill lubricant achieves nearly a 5% reduction per ton.”
Murphy speculates on how this green hot mill lubrication can reduce the carbon footprint of the North American steel industry. He says, “If production reaches 70 million tons, this will cost 54.8 billion pounds of carbon annually in energy use. We estimate that use of the green hot mill lubricant could eliminate 5.48 billion pounds of carbon, leading to an energy reduction of 10%.”
Murphy is looking at future technology that will involve completely water soluble green hot mill lubricants. A formulation will contain non-graphite-based solid film lubricants in combination with extreme pressure/antiwear additives. He says, “This technology has good potential for next generation lubrication but is currently not cost-effective.”
GREEN SPRAY LUBRICANT
Spray lubricants are used to provide corrosion protection and lubrication in commercial and household applications. There are a wide variety of products available in the marketplaces that provide these functions.
LiQuifix has developed what it claims is the world’s first green spray lubricant. Cullinane explains why this product can be designated green. “We strive to insure that our product is minimally harmful to the environment in its production, use and disposal,” he says. “Raw materials that have low negative environmental impact are used to provide these benefits.”
One important aspect with a spray lubricant is minimizing VOCs. Cullinane indicates that his product does not emit any VOCs and also has a flash point good for the environment and user.
Says Cullinane: “We worked with a provider of certification programs known as Scientific Certification Systems to verify that our product meets indoor air quality standards such as Section 01350 of the California Indoor Air Quality Standard, Leadership in Energy Quality and Design, Business & Institutional Furniture Manufacturer’s Association and Collaborative for High Performance Schools. As part of this process, our product was confirmed to emit zero VOCs into the air.”
The spray lubricant has been evaluated in a number of lubricity and corrosion inhibition tests to assess its performance vs. competitive products. Among the tests used are the Falex Pin and Vee Block (ASTM D 3233), Four-Ball Wear (ASTM D 4172) and a one-week corrosion inhibition test conducted in the presence of ultraviolet light and humidity (ASTM D 4587). In all cases, the spray lubricant displayed superior performance.
The spray lubricant also displays the lowest evaporation rate and highest flash point compared to its competitive products. Its flash point is 135 C (275 F), considerably higher than the next highest competitor 80 C (175 F).
“Our product is suitable for any product needing light to medium duty lubrication,” Cullinane says. “Applications include overhead doors, chain conveyors and mechanical switches. We also received certification for USDA H-1, so our product can be used in applications where there is the possibility of incidental food contact (
see Figure 5).”
Figure 5. The green spray lubricant also has achieved certification for USDA H-1, meaning it can be used in food processing applications. (Courtesy of LiQuifix, Inc.)
Positive customer feedback has been received on the spray lubricant from end-users. Cullinane says, “We have been told that less of our product can be used in the same application. For example, the spray lubricant can be applied once per day instead of once per shift. One customer has been able to extend their preventative maintenance schedule from six to eight weeks.”
Cullinane maintains that this product would not be successful if all that could be claimed is its status as a green lubricant. He says, “If our product performed poorly, we would not even have a competitive product. Many of our customers require performance first. They do not have to compromise efficacy to get green.”
GREEN LUBRICANT CONCERNS
A good deal of literature has been published to document negative performance characteristics with green lubricants (
6). Many products have been found to have inferior oxidative stability and low temperature characteristics compared to mineral oil-based products.
DiBiase contends that modification of naturally derived raw materials can boost the performance of green lubricants to levels comparable and even superior to existing products (
see Figure 6). He says, “The key issue with using renewable oils from nature is that they come up a bit short from a performance standpoint. We believe that development of new molecules through use of the biorefinery can change the dynamics. Chemical modification will enhance what nature has provided to enable more efficient and higher-performing lubricants. This can be done without sacrificing the sustainable nature of the renewable raw materials.”
Figure 6. Naturally derived raw materials can be modified to boost the performance of green lubricants while minimizing their negative characteristics. (Courtesy of Elevance Renewable Sciences)
Donaghy contends that a common misconception has occurred that products prepared from renewable feedstocks are inferior to petroleum oil-based lubricants. He says, “There are many materials made from renewable feedstocks available to the market that do not exhibit such vulnerabilities as oxidation. Many of these materials take natural fats and oils, process/refine them and then react them in a manner that provides exceptional thermal stability performance as well as a host of other valuable benefits.”
Donaghy believes that green products such as esters have long been acknowledged to provide outstanding lubrication performance. He indicates that it is up to formulators to be as discriminating in preparing green products as they are in developing traditional petroleum-based lubricants.
Donaghy says, “Esters have been put to good use in many applications, including metalworking fluids and engine oils. It should not be forgotten that there is not a single base fluid or formulation which can be exploited in all lubricant applications when selecting components suited to a given application. There is not one green product but many hundreds, and it is absolutely necessary for the formulator to take the care in determining the best option for a specific application.”
With interest in sustainable or green lubricants growing, the challenge is to better educate lubricant manufacturers about the positive benefits of these materials. Donaghy indicates that economics is the primary driver. “Most consumers focus almost entirely in the upfront cost of the product rather than on the overall cost of utilization of the product,” he says. “Suppliers of green lubricants need to demonstrate that there is an economic driver to go green. Until customers/consumers see the value in a green product or there is a regulatory driver to change, we will see minimal market penetration.”
DiBiase indicates that providing technology that works in a cost/performance fashion is the key to educating customers. Shafer sees a trend of more open-mindedness toward green lubricants in the lubricant industry. “Lubricant manufacturers and end-users understand that alternatives to traditional product technologies are out there, he says. “Manufacturers need better products to work under more rigorous operating conditions, and lubricants derived from green raw materials are now displaying sufficient performance to warrant careful consideration.”
The answer to the question “What is Green?” is still not completely evident but is slowly coming into focus. Lubricants certainly are a key part of the answer.
But the role of lubricants goes beyond the raw materials used in their preparation and their biodegradability. For a lubricant to be green, it must demonstrate in some process a sufficient improvement in productivity and energy reduction to enable the carbon footprint to become either positive or more positive.
The lubricant industry is well positioned to develop and offer greener alternatives to achieve this goal.
REFERENCES
1.
PAS 2050 is available at the BSI Web site: click
here.
2.
Further information can be found
here.
3.
Stubbles, J. (2007), “Steel Manufacturers Association White Paper: Carbon Footprints in U.S. Steelmaking.”
4.
Lawrence, E. (2007), “World Best Practice Energy Intensity Values for Selected Industrial Sectors,” Berkeley National Laboratory, EPA Contract Number:
DE-AC02-05CH11231.
5.
Murphy, J. and Wu, R. (2010), “Reducing Carbon Footprints with Green Hot Mill Lubricants,” Presented at AISTECH 2010 – Iron and Steel Technology Conference, May 2010.
6.
Van Rensselar, J. (2010), “Biobased Lubricants: Gearing up for a Green World,” TLT,
66 (1), pp. 32–41.
Neil Canter heads his own consulting company, Chemical Solutions, in Willow Grove, Pa. You can reach him at neilcanter@comcast.net.