Energy efficiency through precision lubrication
Mike Johnson | TLT Best Practices November 2010
The key is to efficiently manage your human resources.
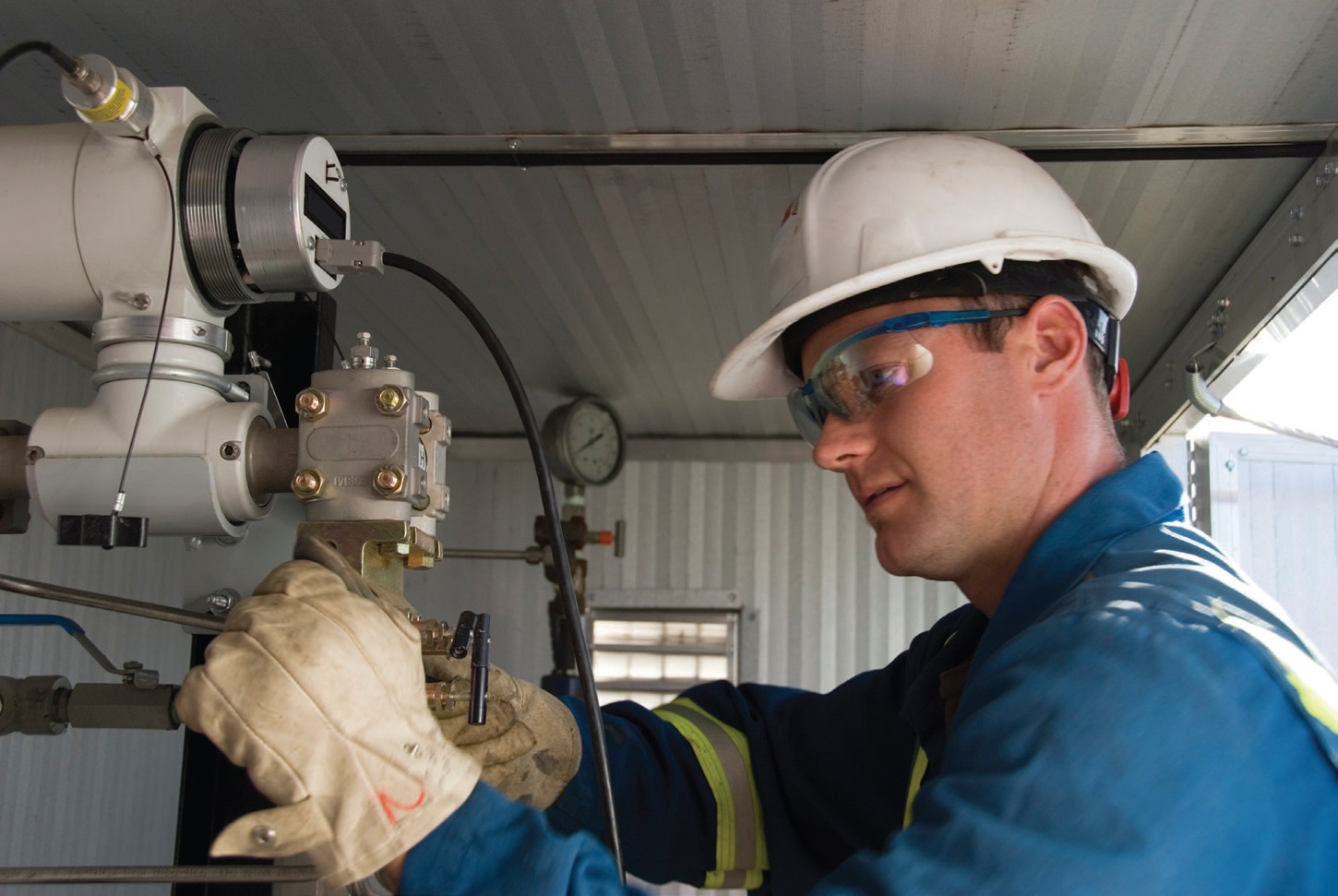
WHAT YOU’LL LEARN
•
The strong theory behind reduced energy consumption through better lubrication practices.
•
How most plants could save huge amounts of money by improving their human energy use.
•
A six-step lubricant evaluation process that reduces energy consumption.
In today’s increasingly competitive manufacturing business, plant managers face the daunting task of finding ways to reduce cost and increase throughput. Energy supply is a significant component of a manufacturer’s cost structure and is relentlessly rising.
Manufacturing processes use energy from such varied sources as coal, natural gas, petroleum and electricity from a generating plant. Some of these energy sources are put to direct use to create other energy sources such as pressurized oil, pressurized air, steam and accelerating chemical reactions.
It is widely known that one of a lubricant’s key responsibilities is surface separation and protection. When properly selected and maintained, the lubricant provides surface clearance that also enables effective energy use. This aspect of machine lubrication is overlooked, as is human energy use. Human energy expended to replace the lubricant represents approximately 5% of maintenance labor, which is only a small portion of the total labor. Much human energy dedicated to lubrication maintenance is squandered through underdeveloped practices.
Opportunities for energy improvement from the machine lubrication process are both obvious and obscure. In this article, we’ll explore some of these opportunities.
TRIBOLOGY—TO COIN A WORD
The word “tribology” was first coined on March 9, 1966, after the publishing of a report by the British Minister of State for Education and Science. The report suggested that the economic value to be derived for the British industry from improvements in lubrication design and practice was worth very big money (
1).
British professor and researcher Dr. H. Peter Jost and his team offered their impression of the potential for improvements in a variety of areas, as shown in Figure 1 (
2). Following this study, Germany, Japan, China and Canada each executed their own studies that have produced insight similar to that of the Jost Report.
Figure 1. Dr. H. Peter Jost’s Projected Economic Benefits Associated With Improved Lubrication Design and Practice.
Jost’s study indicated cost reduction potential for several categories including savings on energy purchases (7.5%), general efficiency (1%) and lubricant purchases (20%). Forty-four years after this study, one could argue that even greater savings potential are available in a modern manufacturing economy from most of these categories.
Following are some arguments to consider for these savings opportunities:
LUBRICATION PROGRAM PROCESS IMPROVEMENTS
There is a large amount of wasted time and effort in plant lubrication programs prior to efficiency analysis and optimization. Practice improvement considerations could include:
•
Using tools and technology (automation) to replace human labor.
•
Optimization of each machine's lubrication requirement.
•
Optimization of each selected lubrication task.
•
Elimination of lubricant replenishment via sealed or unlubricated components.
•
Use of advanced eutectic technologies/materials to limit surface roughness.
LABOR UTILIZATION
A large percentage of lubrication programs operate inefficiently and on auto-pilot. Net labor hours required to fulfill practices tends to be high, and the results often fail to cover the minimum requirements. Labor-saving practices include the following:
15% to 20% (Efficient grease relubrication frequencies). It is common for grease relubrication practices to be inconsistent with component manufacturer’s requirements. Calculation of intervals based on machine operating environment and characteristics is an important first step. Reliability engineers are often surprised to learn that the scheduled frequencies for slow-rotation machines are too short and high-speed (high nDm) applications are too long. Balancing grease relubrication intervals alone may free up labor needed to make other systematic lubrication program improvements.
5% (Use of automation for short-term grease intervals). Grease replacement activities with an interval shorter than seven days should certainly be evaluated for automatic lubrication, either in the form of a single-point grease cup or in the form of simple multi point systems. The choice is based on a cost comparison for installation and long-term maintenance.
20% (Operator-based care, including sump level checks and top-up activities). Level checks and corrections can and should be fulfilled by operators. While it is clear that operators are always busy, part of the operator’s role should be to visit the machines, observe their condition, check the levels and report any observed problems.
10% (Aggressive oil condition control (filtration, cooling)). Lubricant sump change intervals could be safely extended in many applications by a factor of three by simply cooling and cleaning the sumps. The relationship between wear debris, heat and oil oxidation is proven and intuitively obvious. Integrated into the lubricant analysis program, lubricant sump filtration requires effort while the plant is operating but returns labor back to the planning department during outages.
The few items noted above account for 50% of the labor expected for machine lubrication. Freeing this labor for other purposes may require capital (for tools and systems). During the evaluation of practice efficiencies (for a safe return of labor to the maintenance department), the plant engineering department should simultaneously redefine inefficient practices to reflect the best practices of the plant.
MATERIAL CONSERVATION
If the plant engineering department executes the previous improvements, the potential for material conservation (reduced lubricant consumption) already will have been achieved.
Following are three highly visible targets for lubricant consumption improvements:
1.
Compressor Lubricant Usage. Reciprocating cylinder and sliding vane cylinder feed rates are often significantly above OEM suggested values. The excess causes varnish buildup on heat exchangers and coats pipes and air components with excessive oil residue. Synthetic lubricants can be used to optimize the throughput and reduce the risk of varnish accumulation.
2.
Hydraulic and Circulation System Leakage. Hydraulic system designers collectively agree that the majority—perhaps as much as 80%—of hydraulic system leakage is controllable, and much of it is a consequence of poor hydraulic fluid contamination control.
3.
Open Gear Lubricant Application. The AGMA standard 9005-EO2 provides specific guidance on the required volume of open-gear feed per minute of operation for several gear sizes and speeds. Measuring the current feed and judging the potential for reductions in feed is initially a simple mathematical exercise. Judging the adequacy of the feed rate at various steps in the reduction cycle may require the assistance of the lubricant supplier or a knowledgeable technical consultant.
FRICTION REDUCTION
There is a strong theoretical basis for reducing energy consumption by improving lubricant effectiveness. Personal experience suggests that the 7.5% potential for energy reduction noted in the Jost Report may be attainable for some types of applications but not all. The challenges to the reliability engineer is measuring the improvement with some degree of repeatability and overcoming skepticism by engineering and operations managers.
There are a few things to consider:
1.
The Nature of Machine Surfaces. Even the most finely finished machine surfaces are rough. Figure 2 illustrates typical surface profiles for all machined surfaces. Even highly finished bearing element and race surfaces exhibit undulations. As shown in Figure 3, machined surfaces have a wave-like profile. The average of the height of the ridges, value r, differs based on the OEM’s finish technique. Element bearing finishes have maximum surface asperity heights in the .0125 range and average heights in the 0.8 micron range (RMS). Ground gear finishes have surface heights approaching three microns and average heights approaching0. 6 microns (RMS).
Figure 2. All machine surfaces have a significant amount of roughness and potential for frictional energy losses.
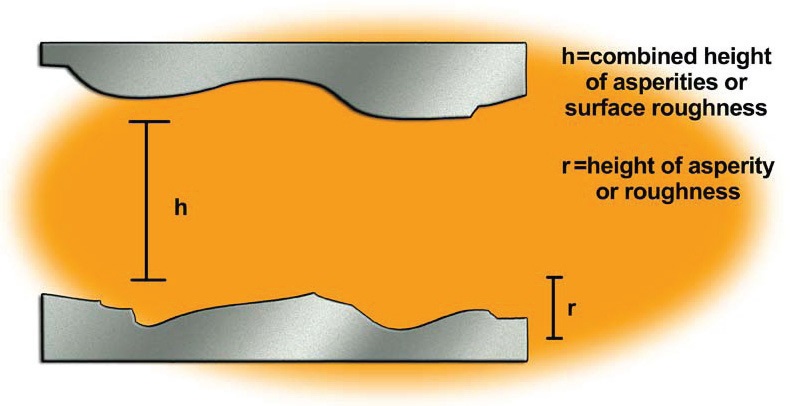
Figure 3. Oil films must be greater than the combined maximum asperity heights to avoid contact and parasitic energy losses.
2.
Separating Machine Surfaces. The dynamic oil film thickness must always be greater than the heights of the combined surfaces in order to avoid surface contact and frictional energy losses. While this might seem all too obvious, this is the essential question at the heart of any calculation to estimate the minimum allowable oil film, which itself greatly influences component life cycle calculations. The ideal condition would be an oil film that is three to five times thicker than the height of the combined surfaces. Component suppliers provide formulas and standardized tools that are useful to establish minimum viscosity operating requirements.
Inadequate film conditions occur as a consequence of changes in load and machine operating temperatures, changes in lubricant condition (particularly contamination with gases or fluids) and accidents in lubricant handling and application, which lead to viscosity errors. These condition changes often occur simultaneously, resulting in film collapse, machine component interaction, greatly increased frictional resistance and greatly increased energy and machine component consumption.
3.
Surface-Protecting Lubricant Additives. OEMs and machine owners collectively recognize that work conditions cause oil films to degrade and fail. Even though the oil film collapses, the machines will continue to run, causing degradation of the machine surfaces. In order to protect the contact area of the lubricated surfaces, lubricant suppliers employ the use of a wide variety of chemicals intended to chemically bond to metal surfaces to create an organometallic boundary layer. Although these tarnish like films are very thin, they do offer protection from excessive wear and prolong machine component life cycles.
Several lubricant manufacturers have made energy conservation claims. These companies create specialized additives that accomplish a variety of end-results from friction reduction to surface improvement. Most of these companies consider their additive technologies to be central to their survival and are tight-lipped about product composition. However, there is no debating that there are additive agents that improve lubricity and reduce energy consumption.
4.
Energy Consumption Influenced by Base Oil Type and Weight. In markets that have federally mandated energy-efficiency requirements such as automotive engines and refrigeration systems, base oil and additive choices are heavily influenced by the material’s energy-efficiency impact. It is widely known that oil viscosity directly influences energy consumption. As viscosity increases, the amount of energy used to overcome viscous drag also increases.
It is less well-known that the type of base oil (molecular composition) influences energy consumption in a couple ways. Some polar stocks, specifically esters and polyglycols, have lubricity properties superior to conventional mineral oil and unconventional hydro cracked stocks. These fluids provide better surface protection with less bulk oil requirement. Consequently, a lower viscosity grade may be adequate to provide similar levels of component protection vs. a mineral oil option. Lower viscosity also enhances flow rate, an important characteristic for heat removal that further enhances energy efficiency (
3).
The combined lubricity and polarity of ester stocks make them an ideal choice for lubricants for reciprocating compressor cylinders. Volumes and viscosities can be reduced, providing reduction in parasitic energy losses from friction and viscous drag. The benefit is pronounced for high-hp machines.
EVALUATION FACTORS
Documenting energy improvement results is not difficult, but it does warrant careful planning and measurements. It is essential to compare similar conditions when making final conclusions.
Following are six factors to consider during the evaluation process:
1.
Identify machines that operate with a narrow range of load, speed and throughput variability. Fluctuations will complicate test cycle comparisons.
2.
Verify that the lubricant in use for the select machine (Product A) is in fact the correct type and grade of product for the application.
3.
Collect process data. At the very minimum, conduct energy readings (amps) for a period of at least 30 days. Also, record ambient temperatures, operating temperatures vs. ambient, machine loads, machine throughput (speed, units of production, RPM, etc.) and/or other process values. Mechanical conditions and changes should also be observed and documented. All maintenance conducted on the machine during the test period should be documented.
4.
Collect mechanical data including sump, motor, drive and bearing temperatures (thermal images), vibration levels and oil analysis data. All of the sample collection methods must be highly repeatable in order for the data to be dependable. Also, a sample set of less than 30 readings can bring the results into question. It is preferable to have too much data rather than too little.
5.
Make the conversion to the alternate product (Product B). Make sure that the sump is completely clear of Product A and that no other mechanical or electrical changes have occurred that might impact the operation of the machine.
6.
Repeat the data collection activity and compare the results.
SUMMARY
Precision lubrication practices support machine reliability interests, produce best use cost and improve labor efficiency. Electrical energy consumption can be reduced through the use of high-performance lubricants and a careful measurement plan.
In addition, human energy consumption can be reduced through careful evaluation and improvement of machine lubrication requirements including the application of high performance lubricants for critical production machines.
REFERENCES
1.
Fitch, J., “Interview with Luminary Professor H. Peter Jost – The Man Who Gave Birth to the Word ‘Tribology,’”
Machinery Lubrication Magazine, January 2006.
2.
Bannister, K. (2007),
Lubrication for Industry, 2nd Edition, Industrial Press, p. 13.
3.
Rudnik, L. and Shubkin, R. (1999),
Synthetic Lubricants and High Performance Functional Fluids, 2nd Edition, Marcel Dekker, p. 88.
Mike Johnson, CLS, CMRP, MLTII, MLA1, is the principal consultant for Advanced Machine Reliability Resources, in Franklin, Tenn. You can reach him at mike.johnson@precisionlubrication.com.