Industrial fluid contaminants and their effect
Mike Johnson | TLT Best Practices September 2009
Air, moisture, heat and particulates are among the enemies threatening the life and performance of your machinery.
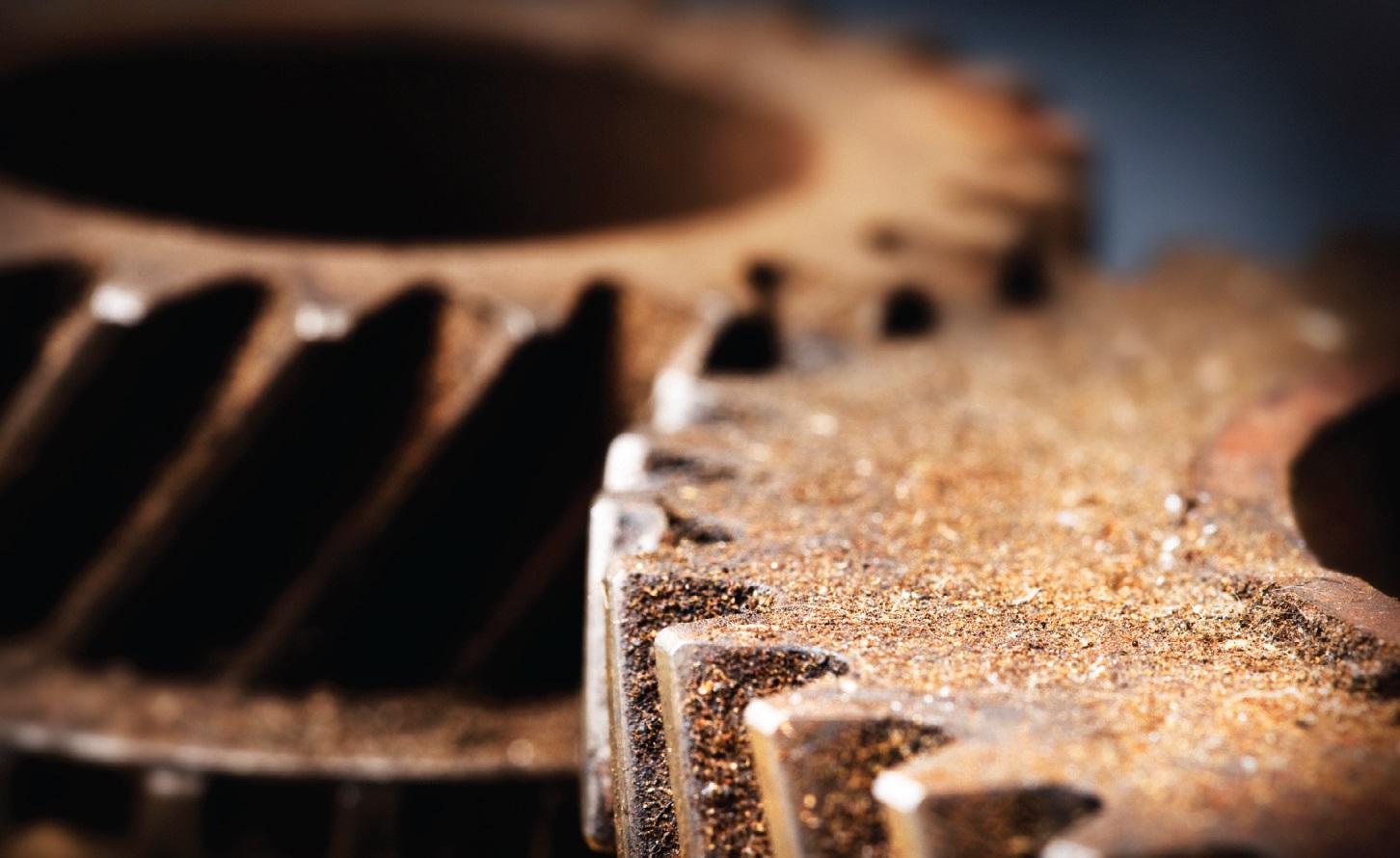
www.canstockphoto.com
KEY CONCEPTS
•
The durability of a tribosystem is limited by the type and concentration of contaminants.
•
Air, water, particulate and heat each represent a destructive threat. When they occur simultaneously, the threat is dramatically increased.
•
Common atmospheric dust particles are harder than machine steel surfaces and are capable of pitting and deforming load surfaces.
Contamination control of sumps is a vital requirement to preserve machine function and component health for all mechanical systems. Without it few systems could achieve their intended purpose or lifecycle (
1).
Destructive contaminants enter lubrication sumps, both oil and grease, and initiate unwelcome chemical and physical reactions and interactions. The products and byproducts of the reactions harm both machine and lubricant in a variety of ways.
A lubricant contaminant is “any amount of material or energy which constitutes a chemically reactive hazard or that mechanically impairs the proper functioning of the equipment and the performance of the system (
2).”
For manufacturing facilities, the most frequently occurring contaminants include:
1.
Air
2.
Moisture
3.
Atmospheric particulate
4.
Machine particulate (metals and their oxides)
5.
Heat
6.
Microbes
7.
Process chemicals
8.
Stored energy.
As it pertains to plant machinery, atmospheric solids, air, heat, moisture and process chemicals are in particular damaging given their common presence in manufacturing environments. Microbial growth is most common to process sumps (for metalworking applications) but can also occur in machine lubricant sumps. Stored energy from potential, kinetic, pressure and tension/strain sources are a threat for structural damage. The degree of threat imposed by the stored energy is a function of the magnitude of the energy potential. Too high or too low a level can represent an unwanted condition to the mechanical system.
This article addresses the common degradation threats to manufacturing machines.
AIR
Air may exist in any one of three different states within a tribosystem:
1.
Free
2.
Dissolved
3.
Entrained (small and large bubbles).
Free air can accumulate in a system during routine work. Free air can become trapped in a hydraulic component or supply line at an elbow or other spaces below the hydraulic oil/lubricant (HOL) level in a mechanical system.
The volume and rate at which air dissolves in the lubricant depends on a variety of factors, including:
•
The specific gravity of the HOL
•
The pressure of the gas or air
•
The pressure of the HOL
•
The temperature of the HOL.
As the HOL temperature and/or pressure increases, the amount of gases dissolved also increases. This is an important consideration for systems that operate at elevated temperatures and pressures.
Air is composed of nitrogen (78%) and oxygen (21%) and other trace gases. Oxygen and nitrogen both can have a strong negative influence on long-term lubricant health. Lubricants dissolve more oxygen than nitrogen for a given increase in temperature or pressure. Lubricant oxidation is any chemical change involving oxygen, and nitration is change involving nitrogen.
The chemical change rate roughly doubles with each 10 C increase in temperature in accordance with the Arrhenius rate rule for organic compounds. The oxidative effect is obvious as the lubricant exceeds 60 C, a common sump temperature. The color and smell of the lubricant change; the lubricant produces long-chain, high-molecular-weight polymers (hydrocarbons) that cause the oil to thicken; sludge deposits form and the disease of self-propagating oil degradation establishes itself in the sump.
Figure 1. Change in air solubility with change in pressure for different types of base fluids (3).
Since it is impossible to prevent air-to-oil contact, it is necessary to equip the machines to tolerate the presence of air and other gases and provide methods to minimize any buildup. System design should enhance air release as quickly as possible. A variety of sump considerations were addressed in the June and August editions of this column.
MOISTURE
Lubricants and hydraulic/circulation oils tend to some degree to absorb moisture from the air. Even the non-polar base oils (API GII, GIII, GIV) that do not support much dissolved water are hygroscopic to a slight extent. Polar additives used with these fluids to create finished lubricants increase the solubility effect. As humidity in the air increases, water concentrations in the lubricant also increase. Any chemicals (soap, other lubricants), water contamination or temperature change that can decrease the surface tension property of the lubricant increases its tendency to hold water. Emulsion-forming tendencies increase when surface tensions decrease (
4).
Water may exist in any one of three different states within a tribosystem:
1.
Free water
2.
Dissolved water
3.
Emulsified water (entrained small and large bubbles).
Free water. This can accumulate in an oil system during routine thermal change (heating and cooling with daytime temperature variations, changes in load and environmental conditions). The water enters the system as vapor with routine thermal cycles. The water vapor in the air space above the oil condenses at the dew point and falls into the oil in the form of water droplets. This is a common cycle that occurs inside machines, storage tanks, drums, small handling containers and any other vessel where the headspace above breathes with atmosphere with temperature changes.
Water is heavier than most lubricant stocks and can fall freely from the lubricant if the conditions are right. Water droplets fall quickly, reside at the lowest portion of the reservoir and remain there until fluid or thermal forces causes the water to mix or dissolve into the lubricant.
Free water allows for microbe growth and poses a chemical threat to the lubricant if it is not removed, but it poses only minimal threat to lubricated components as long as the water does not encounter the component or pass through a load zone within the machine.
Figure 2. Water solubility with changing temperatures (5)
Dissolved water. Individual water molecules can become suspended in the lubricant. As long as the concentration of water molecules is low enough that the molecules don't agglomerate, then the water is considered to be dissolved. Dissolved water cannot be seen but can be measured with common oil analysis techniques.
As the concentration of water molecules increases, and these begin to agglomerate into microscopic water droplets, the droplets can hang in the lubricant, creating a visible cloud. The degree to which the water droplet will hang, or be released to the bottom of the sump, depends on several factors, including:
1.
Type of basestock-polar stocks suspend water more quickly.
2.
Type and concentration of additives-polar additives suspend water more quickly.
3.
Temperature of the lubricant-more water can be suspended as the temperature rises.
4.
Degree of system turbulence-water droplets can be carried in moving oil.
5.
Change in surface tension properties.
6.
Concentration of demulsifier additives in the lubricant.
Emulsified water. As the concentration of suspended water droplets increases, and/or as free water is churned into tiny droplets by the mechanical action of the machine and the cloud becomes visible, then the oil-water is said to be emulsified.
Emulsified water conditions are particularly threatening to mechanical components. Since water has no load-supporting capacity (PV coefficient is very low), when a thin water film or water droplet enters the load zone, the water film collapses and allows surface contact. When this occurs, surface asperities crash together, the oil is burned, the water is vaporized and passes into solution, oxidation byproducts form, wear debris forms and the entire system's health degrades.
It was previously mentioned that temperature has an influence on the lubricant’s tendency to soak up water. As temperature increases, the lubricant sustains more water, and conversely as the temperature decrease water solubility decreases. Fortunately, water also responds to increased temperature loads with a higher evaporation rate. Factors that enhance evaporation can include:
1.
Large air to oil surface area—exposure and separation expand with surface area.
2.
Relative pressure (water boils at lower temperatures at high altitude-low pressure conditions).
3.
High degree of oil movement—short of turbulence.
4.
Sustained, moderately elevated temperature—50 C to 65 C is acceptable.
5.
Good lubricant chemical health—exhibited by high interfacial tension.
WATER MANAGEMENT
Filter manufacturers have devised a variety of moisture-removal systems around these physical response characteristics. One of the most effective methods to drive water off is the vacuum dehydration filter method.
In vacuum dehydration-type filtration, the lubricant is pumped into a vacuum chamber where the pressure is decreased (25 inches HG) and the temperature is raised to a level that will allow water to boil (133 F/56 C) at a low temperature. More heat and lower pressure decreases the temperature of evaporation. Vacuum dehydrators are used in large systems and systems with a high water contamination risk. Paper machines, turbines, hydraulic systems that operate in humid environments, any large sumps that are operated intermittently and any systems that have old water type heat exchangers would be good candidates for this technology.
Absorbent filter elements incorporate super-absorbent materials into depth media (elements) for removal of smaller quantities of water from smaller sumps. As the absorbent becomes water soaked, the pressure differential increases. At a high differential pressure, the element would be replaced.
Centrifugal separation forces can cause emulsions to break and the water to separate by weight. Differences in the specific gravity (weight) of the substances in the lubricant are amplified when the substances are subject to high centrifugal forces.
Force is applied by spinning the fluids at extremely high speeds in a cone-shaped barrel called a centrifuge. High rotational speeds create high gravitational forces (high Gs). As the G-forces increase, the weight differences between the lubricant, water and contaminant increase as well, and the water begins to break free from the oil and agglomerate. Eventually water drops and separates from the lubricant.
SOLID PARTICLES
Particles are a threat to the component surfaces and control mechanisms in all tribosystems. Microscopic particles promote micropitting, fatigue and three-body wear in proportion to their concentration in the oil.
Particle density, hardness, shape and concentration each influence particle destructive potential. Particle density is the mass of a particle for a given unit volume. The unit expressed as grams per cubic centimeter is equivalent to specific gravity. The density of a particle can influence destructive potential through risk of impingement and settling speed.
Resistance to surface abrasion is used to depict particle hardness. A particle that is harder than a machine surface can abrade, scratch and deform machine surfaces. Hardness is also referred to as toughness or the property of the material that allows it to absorb energy when stressed beyond its elastic limit. The Brinell and Mohs scales, as shown in Figure 3, rate the hardness of various materials, including common atmospheric contaminants.
Figure 3. A comparison of Brinell and Mohs hardness scales for common elements and compounds.
Particle shape is characterized by two factors. First, the extent to which the particle approaches a predefined form (sphere, cube, tetrahedron, etc.). Second, the relative proportions of the particle. Proportions of key interest are particle (
6) width (breadth, surface area) to thickness, and length to width. Particle shape has tremendous influence on accurate estimation of particle concentration. Since naturally occurring particles are irregular and difficult to accurately gauge, particle estimations are done by measuring the dimension of many particles in random orientation, producing statistical estimates of the sizes of particles in large populations.
Particle concentration is probably the most clearly understood parameter. There are several methods useful to gauge particle concentrations, but the most widely used is the International Standards Organization fluid cleanliness code (ISO 4406:99). This three-part system indicates concentrations of particles at and greater than four-, six- and 14-micron sizes. A thorough review of this standard will be provided in a later article.
The solid particle sizes that impose the greatest threat to machine surfaces, those that are small enough to get between the surfaces and bridge the oil film gap, are well below that which can be seen without the use of a microscope. Add to this the fact that particles become more difficult to crush as the size decreases. Particles that are 1-3 microns are particularly tough and particularly destructive to machine surfaces.
Atmospheric dust can change the surface profile of the machine component if the hardness of the particle is greater than that of the component steel. Silica-laden atmospheric dust can have a greater hardness rating than many machine steels. When dust particles fracture to a size small enough to enter the dynamic clearances (± 2 microns) at component contact interfaces, these hard, tough particles can cause jagged indentions in the surface profiles, as shown in Figure 4.
Figure 4. Microphotograph of hard particle indentions of machine surfaces. (Courtesy of Lubcon, GmbH)
Higher particle counts mean higher wear rates for all types of lubricated components. As wear increases, the catalytic effect of iron and copper debris accelerates chemical reactions and increases the rate of aging.
HEAT CONTROL
Poor thermal conditions represent a contaminant. Too much or too little heat can be a problem. An ideal sump temperature is 50 C to 60 C. Within this range, water is managed by evaporation, particles settle quickly, the oil flows freely and the rate of oxidation is low. When wear metals and water increase together, the lubricant health degrades rapidly.
Figure 5. Contaminant effect on oxidation rate on mineral oil stocks (7)
Heat can be removed from the system by constantly circulating the lubricant out of the tank through a heat exchanger (fluid version of an air conditioner) and placing cooler oil back into the tank. Where large amounts of heat removal are required, the necessary equipment may cost thousands of dollars.
Heat can be introduced to a system in the same way or may be added to a system by use of a heat conductor mounted in the tank in an area of high flow.
SUMMARY
Contaminant monitoring and control is of paramount importance to machine productivity. Air, water, particulate and heat are dominant contamination threats to industrial machines.
Each contaminant poses a discrete threat to oil and components. When contaminant threats overlap, the rate of stress dramatically increases, raising the risk of failure for the oil/lubricant, the machine components and eventually machine capacity.
REFERENCES
1.
Fitch, E.C.
Fluid Contamination Control, FES, Inc., Stillwater, Okla., p. 1.
2.
Fitch, E.C.
Proactive Maintenance for Mechanical Systems, Second Edition, FES, Inc., Stillwater, Okla., p. 19.
3.
IBID 1, p. 20.
4.
Fitch, E.C.
Fluid Contamination Control, Chapter 1, Section 3, FES, Inc., Stillwater, Okla.
5.
IBID 3.
6.
IBID 3, p. 10.
7.
Fitch, E.C. (1992), Proactive Maintenance for Mechanical Systems, Second Edition, FES, Inc., Stillwater, Okla., p. 105.
Mike Johnson, CLS, CMRP, MLT, is the principal consultant for Advanced Machine Reliability Resources, in Franklin, Tenn. You can reach him at mike.johnson@precisionlubrication.com.